- Home
- Classes
- Physics 150 – Nuclear Weapons – Physics and Policy – Winter 2025
- Astro 1 Spring 2024
- INT 86TN Planetary Defense – Spring 2024
- Symmetry & Aesthetics in Contemporary Physics
- INT 184 – PL Interdisciplinary Honors Seminar – Weapons of Mass Destruction – Spring 2013
- Physics 150 – Modern Design and Fab – Fall 2019
- Physics 134 – Observational Astrophysics – Fall 2024
- Physics 4 – Winter 2023
- Physics 141 – Optics – Spring 2019
- Physics 145L – Astrophysics Research
- Physics 199 – Independent Research
- Projects
- Interdisciplinary Center for Interstellar Exploration (iC)
- NASA Watts on the Moon
- Extrasolar Travelers
- CMB-S4 – Ground Based CMB Cosmology Program
- Wafer Scale Spacecraft
- PI-Multimodal Planetary Defense
- Lunar Rover Project
- DE-STAR
- Starlight
- GreenPol – CMB Cosmology
- PLANCK
- Starshot
- SETI
- Small Projects
- Previous Projects
- Facilities
- People
- Net
- Outreach
- Media Links
- NASA Space Grant Student Scholarships
- Bright Stars – VoH
- ARC – Undergraduate Aerospace Research Coop
- POINT – Undergraduate Physics Organization for Innovation and Technology
- All Sky Camera Project
- Education and Public Outreach
- Gaucho Rocket Project
- Undergrad Research Opportunities
- The Space Race
- Misc
- Papers
DE-STAR
Directed Energy Planetary Defense
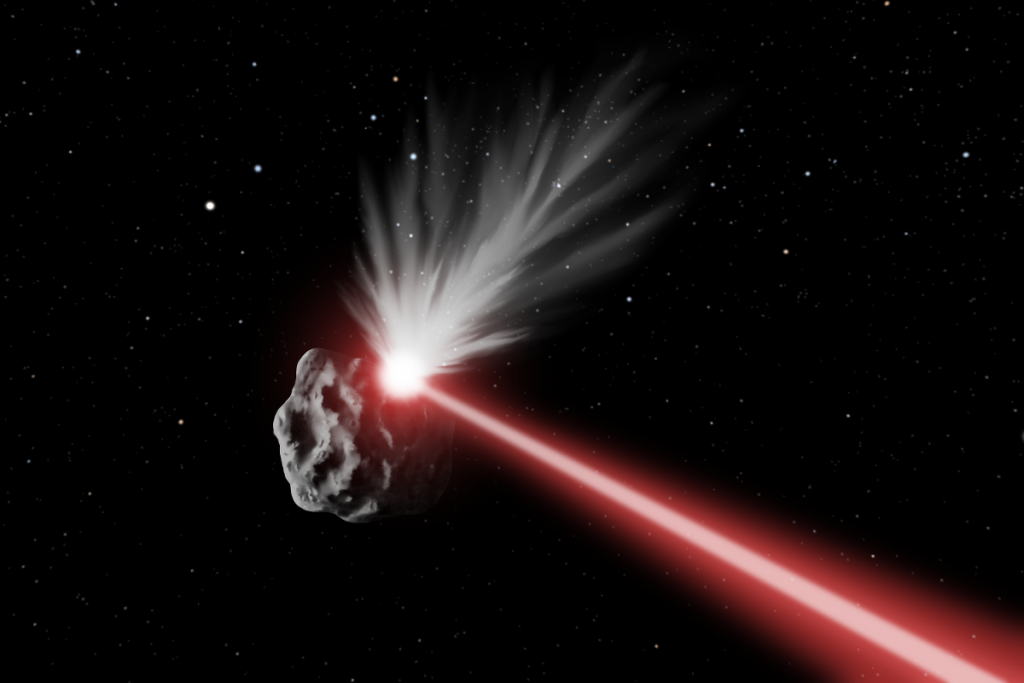
Planetary defense by high-powered laser: material ablated off a would-be impactor alters its trajectory by conservation of momentum to avert an impact. (Q. Zhang)
DE-STAR or Directed Energy System for Targeting of Asteroids and exploRation is a proposed system to deflect asteroids, comets, and other near-Earth objects (NEO) that pose a credible risk of impact. The objects that cross Earth’s orbit, even relatively small ones, can still have a devastating effect. We propose an orbital planetary defense system capable of heating the surface of potentially hazardous objects to the point of vaporization. DE-STAR is a modular phased array of kilowatt class lasers powered by photovoltaics.
We consider two classes of systems:
- large “stand-off” DE-STAR arrays, which remain in Earth orbit and deflect the target from afar, and
- much smaller “stand-on” DE-STARLITE systems which travel to and deflect from alongside the target
The modular design allows for incremental development and test, lowering cost, minimizing risk, and allowing for technological co-development. While DE-STAR is designed as a stand-off system (able to accomplish a task from afar), DE-STARLITE is a much smaller version which is deployable on a single launcher but still capable of mitigating large asteroids given sufficient warning.
In both cases, highly-focused energy raises the temperature of a spot on the target’s surface to ~3000 K, allowing direct vaporization and ejection of surface material altering the asteroid’s or comet’s orbit. Ideal DE-STAR systems can simultaneously engage multiple targets.
Additional applications of these arrays include space debris mitigation, powering or recharging of distant probes, standoff power to remote facilities, standoff photon drive propulsion of small spacecraft that can achieve relativistic speeds (see DEEP-IN), composition analysis of remote objects including asteroids, and many others. The implications for SETI and ultra long range beacons extending even beyond our galaxy are also discussed.
Recent Publications
List of recent Directed Energy related publications: DE_STAR_and_related_References
Orbital Deflection of Comets by Directed Energy – Astronomical Journal (AJ) (2019)
by Q. Zhang, P.M. Lubin and G. B. Hughes
http://arxiv.org/abs/1904.12850
Directed Energy Missions for Planetary Defense – Adv Space Res 2016
Advances in Space Research (ASR) – Volume 58, Issue 6, 15 September 2016, Pages 1093-1116
http://arxiv.org/abs/1604.03511
Orbital Simulations on Deflecting Near-Earth Objects by Directed Energy (2016)
by Q. Zhang, K. J. Walsh, C. Melis, G. B. Hughes, P. M. Lubin
This paper discusses the use of numerical simulations to evaluate the effectiveness of a range of directed energy systems on a range of potential targets, focusing on asteroids but also with a brief discussion on comets.
Preprint: arXiv:1601.03690
Publications of the Astronomical Society of the Pacific:
Volume 128, Number 962, Article 045001
Directed Energy Planetary Defense (Book Chapter – 2015)
by P. Lubin, G. B. Hughes
Chapter in Cosmic Hazards and Planetary Defense – Springer (We receive no funds from this)
Download Chapter (PDF) – Pages 941-991
Toward directed energy planetary defense (2014)
by P. Lubin, G. B. Hughes, J. Bible, J. Bublitz, J. Arriola, C. Motta, J. Suen, I. Johansson, J. Riley, N. Sarvian, D. Clayton-Warwick, J. Wu, A. Milich, M. Oleson, M. Pryor, P. Krogen, M. Kangas, H. O’Neill
Optical Engineering: Toward directed energy planetary defense (PDF)
Conferences & Proceedings
SPIE Optics + Photonics – San Diego – August, 2016
Hughes et al. “Remote laser evaporative molecular absorption spectroscopy”: Paper
Madajian et al. “Comet deflection by directed energy: a finite element analysis”: Paper
Zhang et al. “Simulations of directed energy comet deflection”: Paper
Macasaet et al. “Target tracking and pointing for arrays of phase-locked lasers”: Paper
SPIE Optics + Photonics – San Diego – August, 2015
Hughes et al. “Stand-off molecular composition analysis”: Paper
Zhang et al. “Orbital simulations on the deflection of Near Earth Objects by directed energy”: Paper, Presentation
Brashears et al. “Directed Energy Deflection Laboratory Measurements”: Paper
Griswold, Madajian et al. “Simulations of directed energy thrust on rotating asteroids”: Paper
Steffanic et al. “Local phase control for a planar array of fiber laser amplifiers”: Paper
Research Mentorship Program – UCSB – July, 2015
Georgieva et al. “Using a Directed Energy System to Deflect Asteroids”: Paper, Poster
Gilkes et al. “De-Spinning Asteroids: Using Laser Ablation to Manipulate Asteroid Motion”: Paper, Poster
Silverstein et al. “Space Debris Mitigation Utilizing Laser Ablation”: Paper, Poster
Hypervelocity Impact Symposium – Boulder, CO – April, 2015
Zhang et al. Orbital Simulations for Directed Energy Deflection of Near-Earth Asteroids
Planetary Defense Conference – PDC – Frascati, Italy – April, 2015
Lubin et al. Effective Planetary Defense using Directed Energy
Brashears et al. “Directed Energy Deflection Laboratory Measurements”: Paper, Poster
News & Multimedia
The Asteroid Hunters (Popular Mechanics – Nov, 2015)
A popular overview of background and current detection and mitigation work.
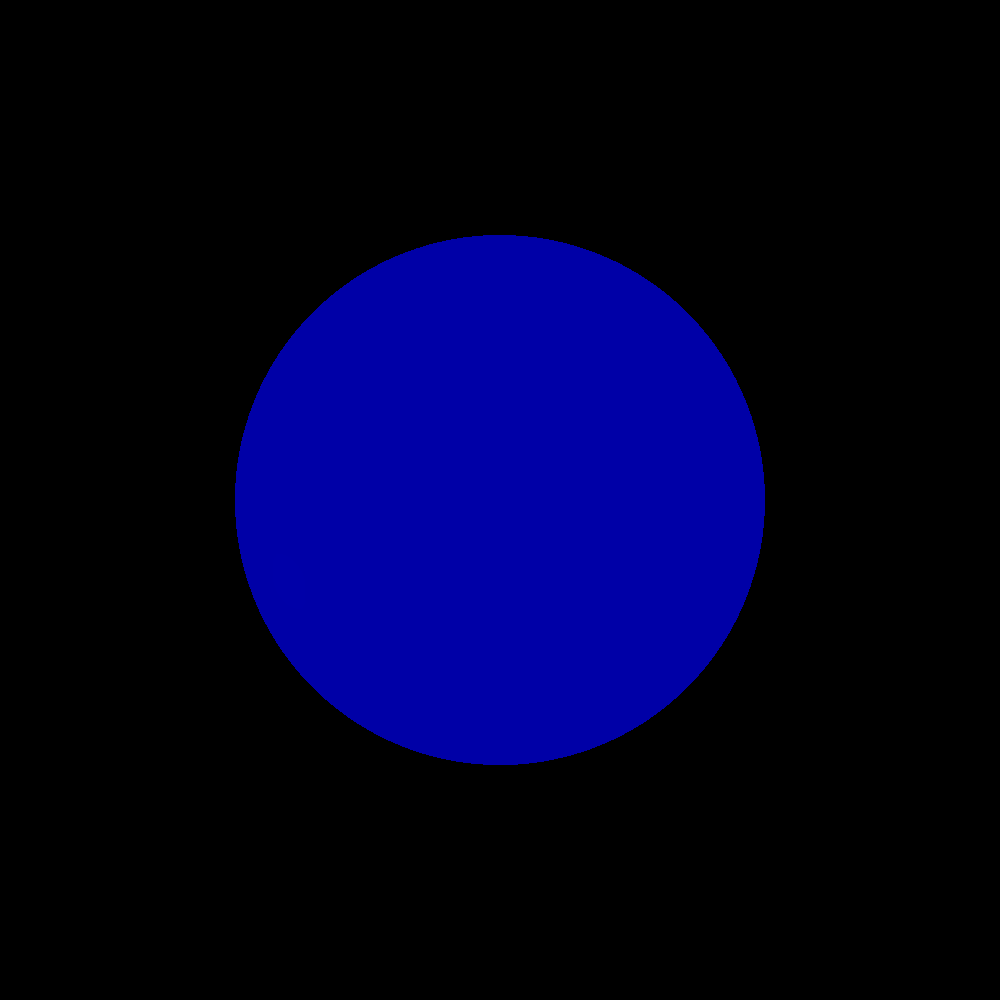
FEA simulation of directed energy on a 1m diameter SiO2 rotating asteroid with a 100s period over 4 days using a 1 MW laser. Note that the small asteroid size and high speeds are due to numerical limitations and are not indicative of the project’s scale. Made with Comsol by Wu, Johansson, Griswold, and Madajian
Vacuum Chamber Demos
Rotation and Derotation – July, 2015
Planetary Defense Conference – April, 2015
Laser at >10 MW/m2 (see Brashears et al.)
A
PDC April 2015 Simulated Threat (click to expand)
A hypothetical threat from a large asteroid was presented at the Planetary Defense Conference in Frascati, Italy in April 2015 (see PDC 2015 threat simulation details – PDF). Orbital simulation are done with a 3 body numerical solver and the results are compared to analytic approximations that are sometimes used (the 3 delta approximation). The numerical simulations are the proper way to look at a detailed mission while the analytic approximations are used for quick rough mission designs.
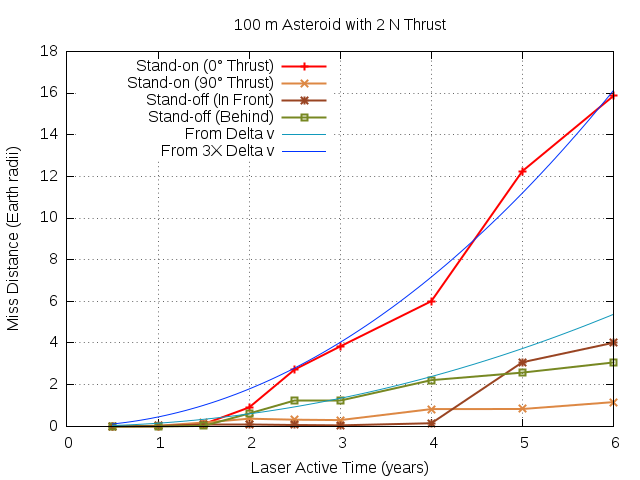
100m-2N.mvt
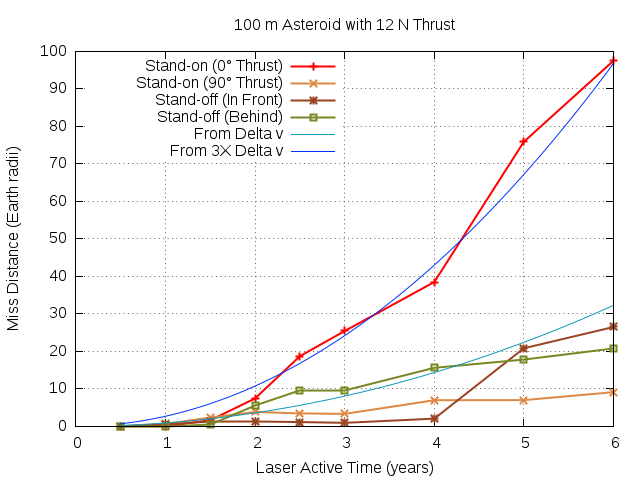
100m-12N.mvt
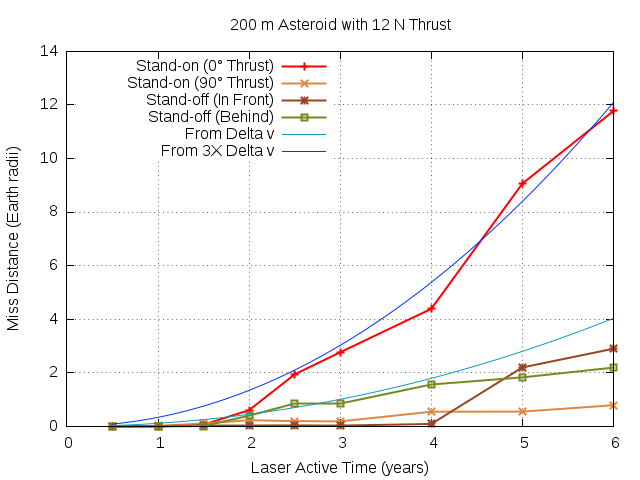
200m-12N.mvt
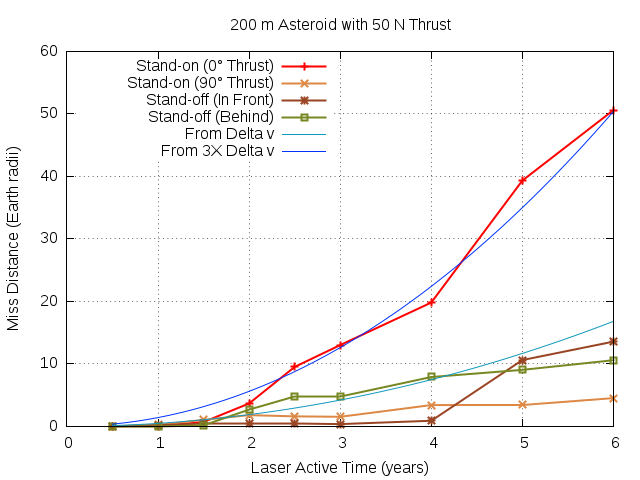
200m-50N.mvt
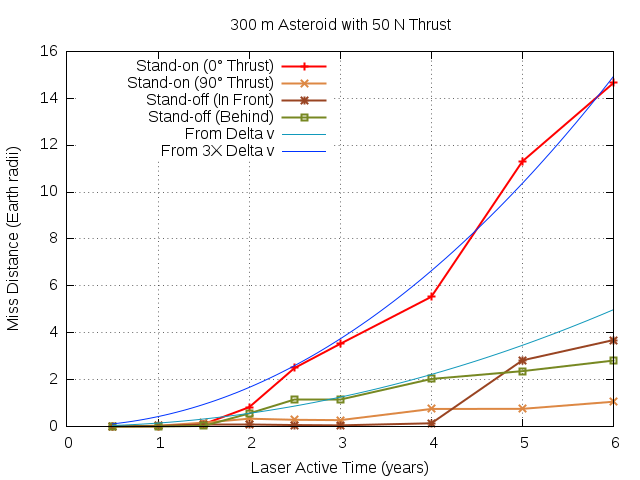
300m-50N.mvt
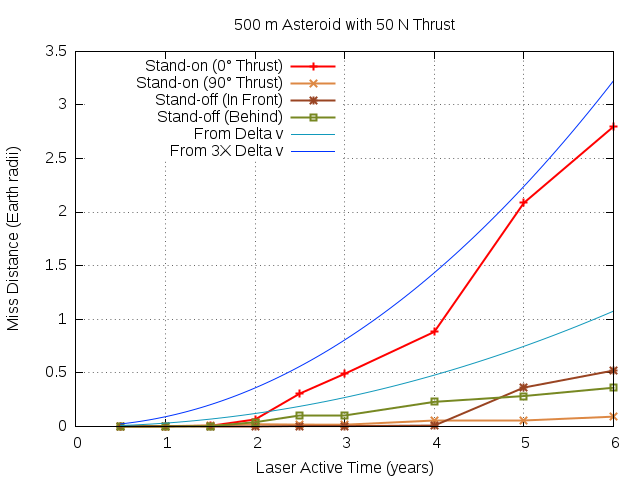
500m-50N.mvt
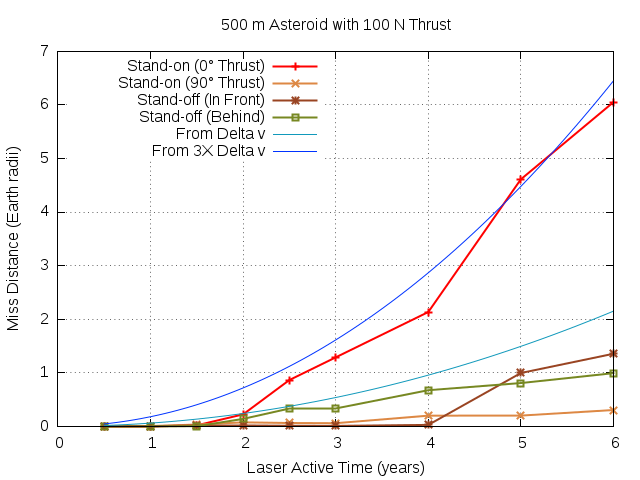
500m-100N.mvt
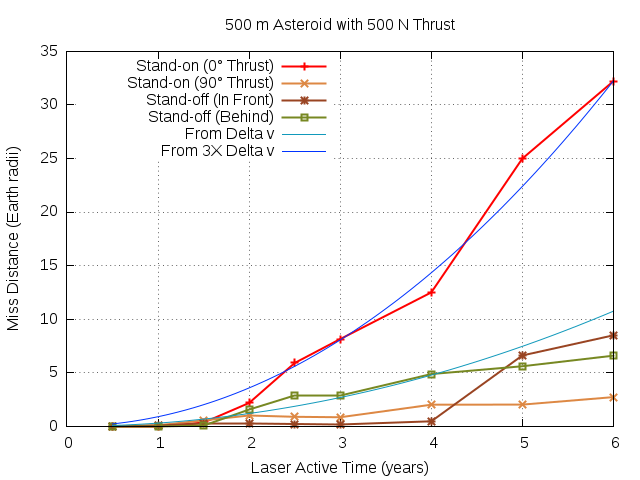
500m-500N.mvt
Suppose we send a DE-STARLITE mission to an asteroid and it arrives at the asteroid 4 years before impact (when the asteroid is ~2.9 au from the Earth). How far will the asteroid be deflected? Here’s a comparison of a 100 m, 200 m and a 300 m diameter asteroid with a 12 N thrust (~ 100-200 kW laser). As can be seen even large asteroids can be effectively deflected even with modest DE-STARLITE missions. If we begin the interdiction process even earlier the laser power requirements are reduced or if larger power is used even short interdiction times are feasible. See our papers for more detailed mission discussions.
SPIE Optics + Photonics – San Diego – August, 2014
Kosmo et al. DE-STARLITE – A Directed Energy Planetary Defense Mission
Johansson et al. Effects of asteroid rotation on directed energy deflection
Riley et al. Directed energy active illumination for near-Earth object detection
Hughes et al. Optical modeling for a laser phased-array directed energy system
SPIE Optics + Photonics – San Diego – August, 2013
Lubin et al. Directed Energy Planetary Defense (plenary)
Hughes et al. DE-STAR: Phased-Array Laser Technology for Planetary Defense and Other Scientific Purposes
Bible et al. Relativistic Propulsion Using Directed Energy
Plentary talk by P. Lubin:
SETI – February 2014
SETI Big Picture Science Radio Show Interview: Space For Everyone: Philip Lubin by Niederhoff
Presentation: Directed Energy for Planetary Defense and Implication for Searches for Advanced Civilizations
DE-STAR: A Planetary Defense and Exploration System
News Article Asteroid-zapping lasers step out of science fiction by Burkhart
Video Interview Philip Lubin: A space-based array for planetary defense by Donnelly and Probasco
Miscellaneous Videos
Laboratory tests of high efficiency 19 element laser at 808 nm focused onto a Basalt target at a flux of about 20 MW/m2. Max spot temperature is mass ejection limited at about 2600-3000K.
Physics based simulation of laser interaction with asteroid Apophis (325 m diameter) at 1 AU. Made by Caio Motta with Cinema 4D Studio donated by MAXON Computer.
Plume ejecta speeds are approximately 1 km/s. Asteroid composition is typical high temperature rocky material (Si, Al, Fe, Mg oxides etc) with a spot temperature that is mass ejection limited at about 3000 K for this example compound.
We gratefully acknowledge support from the NASA California Space Grant Consortium.