- Home
- Classes
- Physics 150 – Nuclear Weapons – Physics and Policy – Winter 2025
- Astro 1 Spring 2024
- INT 86TN Planetary Defense – Spring 2024
- Symmetry & Aesthetics in Contemporary Physics
- INT 184 – PL Interdisciplinary Honors Seminar – Weapons of Mass Destruction – Spring 2013
- Physics 150 – Modern Design and Fab – Fall 2019
- Physics 134 – Observational Astrophysics – Fall 2024
- Physics 4 – Winter 2023
- Physics 141 – Optics – Spring 2019
- Physics 145L – Astrophysics Research
- Physics 199 – Independent Research
- Projects
- Interdisciplinary Center for Interstellar Exploration (iC)
- NASA Watts on the Moon
- Extrasolar Travelers
- CMB-S4 – Ground Based CMB Cosmology Program
- Wafer Scale Spacecraft
- PI-Multimodal Planetary Defense
- Lunar Rover Project
- DE-STAR
- Starlight
- GreenPol – CMB Cosmology
- PLANCK
- Starshot
- SETI
- Small Projects
- Previous Projects
- Facilities
- People
- Net
- Outreach
- Media Links
- NASA Space Grant Student Scholarships
- Bright Stars – VoH
- ARC – Undergraduate Aerospace Research Coop
- POINT – Undergraduate Physics Organization for Innovation and Technology
- All Sky Camera Project
- Education and Public Outreach
- Gaucho Rocket Project
- Undergrad Research Opportunities
- The Space Race
- Misc
- Papers
GreenPol – CMB Cosmology
Greenland Polarization Experiment
Project Overview
GreenPol will use a microwave telescope designed to measure the polarization of emission from our galaxy at frequencies of 10, 15, 20, 30 & 44 GHz at Summit Station in Greenland. The purpose of GreenPol is to understand the galactic emission in order to better understand and remove this structure from deep Cosmic Microwave Background (CMB) anisotropy and polarization maps, such as those generated by the Planck mission. By removing the polarized contamination from our galaxy, researchers will be able to generate more sensitive CMB maps, which will help them have a better understanding of the early universe, particularly in the search for primordial gravitational waves, which if found would be strong evidence for the theory of inflation.
B-mode Polarization Forecasts for GreenPol
Fuskeland et al, Astronomy and Astrophysics (A&A) 684, A128, 2024
https://doi.org/10.1051/0004-6361/202347393
https://arxiv.org/abs/2306.13043
The Cosmic Microwave Background:
The Cosmic Microwave Background (CMB) is currently the earliest picture of our universe that we can detect. The early universe consisted of very hot dense hydrogen plasma, which was opaque, meaning photons were not able to propagate. As the universe cooled and expanded, the hydrogen plasma became hydrogen atoms making the universe transparent, allowing the photons to propagate. We can still observe these photons today and it is what we call the CMB. Fluctuations or anisotropies observed in the CMB give cosmologists information about density fluctuations in the early universe plasma as well as other conditions at that time. The polarization of the CMB may hold clues of primordial gravitational waves, however contamination from polarized sources in our galaxy must be removed first. In our frequency range the primary sources of polarized microwaves from our galaxy come from synchrotron radiation, which is caused by charged particles moving through the galactic magnetic fields at speeds close to the speed of light, free-free emissions from electron-ion scattering, thermal dust emission from heated interstellar dust grains, and possibly emissions from spinning dust.
Why GreenPol?
The millimeter wavelength sky is critical for understanding cosmological foregrounds in order to remove the galactic signature from the cosmological signature. This is especially important in searching for the gravity wave signature from the proposed inflationary era from polarization in the Cosmic Microwave Background (CMB). Our galaxy has two primary and very different emission mechanisms, namely synchrotron emission from high energy Cosmic Rays that dominates below 100 GHz and dust emission from heated interstellar dust grains that dominates above 100 GHz. It is critical that we have a series of very sensitive maps from 10-100 GHz to understand the synchrotron component of our galaxy to combine with the Planck high frequency dust maps from 100-900 GHz. The current WMAP and Planck maps at 23, 30, 40 and 70 GHz are insufficient, especially in polarization which neither satellite was specifically designed to measure and for which there is both insufficient sensitivity and serious systematic concerns. There are two major goals for our longer term effort in Greenland. One is to study the galactic foregrounds by measuring over about 50% of the sky, doing for synchrotron what Planck has done for dust, AND to feed these maps and understanding into the deep cosmological maps we will make from Greenland at 10-44 GHz and eventually 100 GHz to search for evidence of gravitational waves from the early universe.
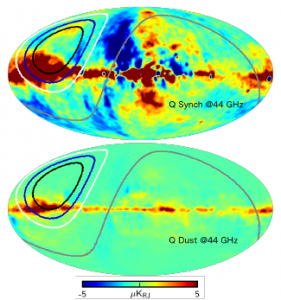
The lines show the sky region covered by a 10 degree (black), 20 degree (blue), 30 degree (white), and 65 degree (gray) opening angle
In order to do the latter (search for gravitational waves) we MUST do the former (characterize the galactic foregrounds) as so poignantly been shown by the recent Planck release. Greenland is one of the best observing sites in the world, particularly with respect to precipitable water vapor, and allows coverage of the northern hemisphere, which is less contaminated by the galaxy and is complimentary to southern hemisphere measurements at higher frequencies that study dust contaminated frequencies.
A critical factor that is new is that the Planck data has recently shown that the higher frequency (about 100 GHz) polarization measurements are heavily contaminated by a much more complex dust emission than we had anticipated and at a higher level than anticipated. Since the atmosphere is quite absorptive above 100 GHz and essentially opaque above 300 GHz, with only a few observing frequency windows, in order to fully characterize the dust emission space based systems are needed. On the other hand the lower frequencies below 100 GHz are essentially completely open to observation from the ground. This allows us to make much more detailed measurements of the galactic contamination from ground based measurements. Additionally the advantage of going into space compared to ground is only about a factor of 2 in system noise for the critical bands while at higher frequencies ground observations are much less sensitive than space both due to the increased opacity of the atmosphere and the decreased flux from the CMB at higher frequencies. These two effects give us a strong science case to push to lower frequencies and to use the high altitude site in Greenland (Summit site) as an observing site. Another critical factor that favors observing at lower frequencies is that the technology we will use to make these measurements uses detectors that are extremely linear compared to the bolometers used at higher frequencies. Thus atmospheric perturbations from water vapor fluctuations and pressure waves will be largely cancelled out to a much higher degree than bolometer based measurements, allowing us to get polarization information on larger angular scales that is critical to understanding inflation.
Greenland Summit Station PWV analysis: http://128.111.23.62/wordpress/wp-content/uploads/2010/08/T-TST-REG-04-2013-00044.R1_final.pdf
B mode Polarization forecasts for GreenPol
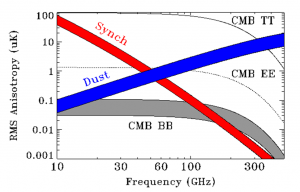
RMS anisotropy of CMB compared to Galactic foreground emission (Rayleigh Jeans Temp). Grey is the predicted range for inflation for simple models. The actual signal level is unknown. The red and blue bands show typical high-latitude emission from polarized synchrotron and dust emission within the Galaxy.
Gravitational waves from inflation acting as tensor perturbations can produce B-mode polarization (named for its divergence free nature as is the case with magnetic fields) when interacting with light. If inflation is correct we should then be able to detect B-modes in the CMB. The amplitude of B-modes is parameterized with what is known as the tensor to scalar ratio r, currently measured to be r < 0.07 at 95% confidence by the BICEP2/Keck collaboration. If there were no gravity waves in the early universe then we would only see scalar perturbations in the CMB and r would be zero. As it stands, the small upper bound on r illustrates the need to very accurately characterize galactic foreground emission.
The following data is taken from a soon to be published paper by Unni Fuskeland et al. at the Institute of Theoretical Astrophysics at University of Oslo which presents tensor to scalar ratio forecasts for GreenPol.
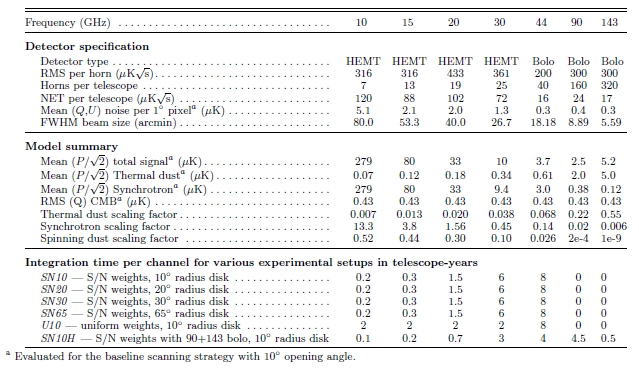
Summary of GreenPol instrument properties (top section), model parameters (middle section), and experimental setups (bottom section) for our proposed experiment. Temperatures are given in thermodynamic units.
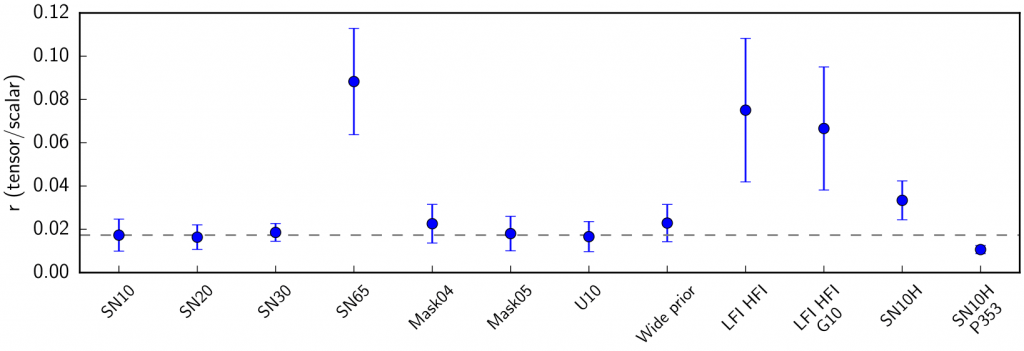
Tensor to scalar ratio r for various experimental set ups at the 95% confidence limit. Each point represents the mean of the 95% confidence limits evaluated from 20 simulations. The error bar indicates the 68% region among the same simulations. The horizontal gray dashed line is the value of the baseline configuration SN10.
The experimental setup for each point in the above plot is as follows. Unless stated otherwise, all set ups follow the baseline configuration (SN10) of 10-44GHz GreenPol channels + 100-353 GHz Planck channles at a 10 degree opening angle.
- SN10, SN20, SN30, & SN65 – opening angle of 10, 20, 30, & 65 degrees. Each frequency channel is weighted by its signal to noise ratio
- Mask04 – Pixels are masked by thresholding the Planck synchrotron polarization, leaving a full sky fraction of 0.73
- Mask05 – Pixels are masked by thresholding the CMB posterior RMS map at 0.4 uK
- U10 – Uniform weighting across frequency channels
- Wide prior – Gaussian priors on the spectral parameters for synchrotron and dust are tripled
- LFI HFI – Planck LFI + HFI channels only
- LFI HFI G10 – Planck LFI + HFI + 10GHz GreenPol channel
- SN10H – 10-44GHz + 90, 143 GHz GreenPol channels
- SN10H P353 – 10-143 GHz GreenPol channels + Planck 353 GHz channel
Combing Planck HFI observations on polarized thermal dust emission with the proposed experiment, our collaborators from the Institute of Theoretical Astrophysics at University of Oslo estimate a limit of r < 0.02 at 95% confidence for the baseline configuration (SN10). This limit is derived from ideal and simplified simulations which account for foregrounds (thermal dust and synchrotron) and white noise, but not instrument systematics. Variations in a number of experimental parameters such as sky coverage, detector weighting, and foreground priors, have little effect on this limit, making it very robust. GreenPol therefore has the potential to provide the most sensitive low frequency CMB polarization measurements of the northern galactic hemisphere in the foreseeable future.
Greenland Summer 2016:
A brief RFI and site survey was done in the summer of 2016 in preparation for deployment.
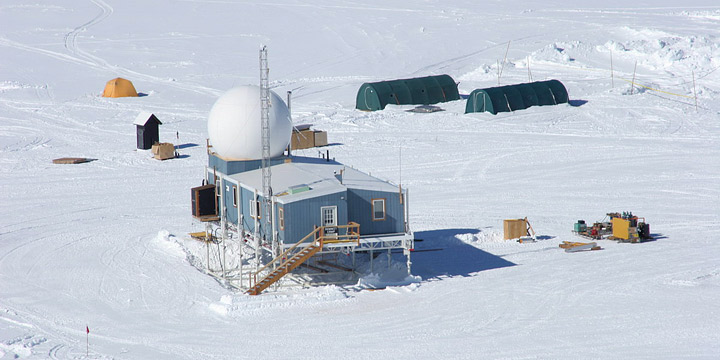
Summit Station
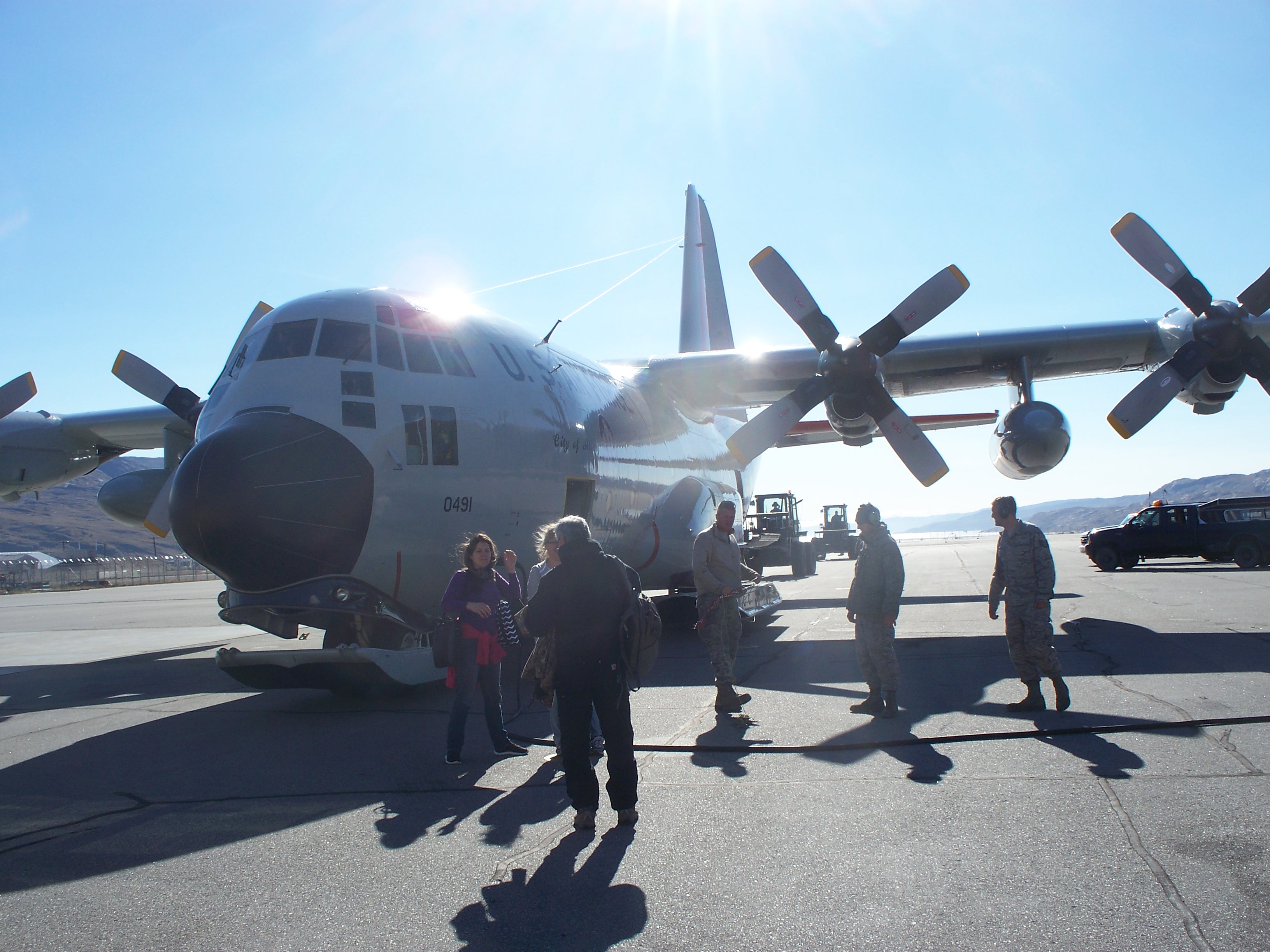
Plane From Kangerlussuaq to Summit
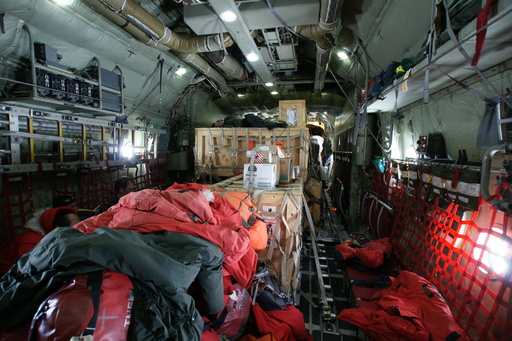
Plane Interior
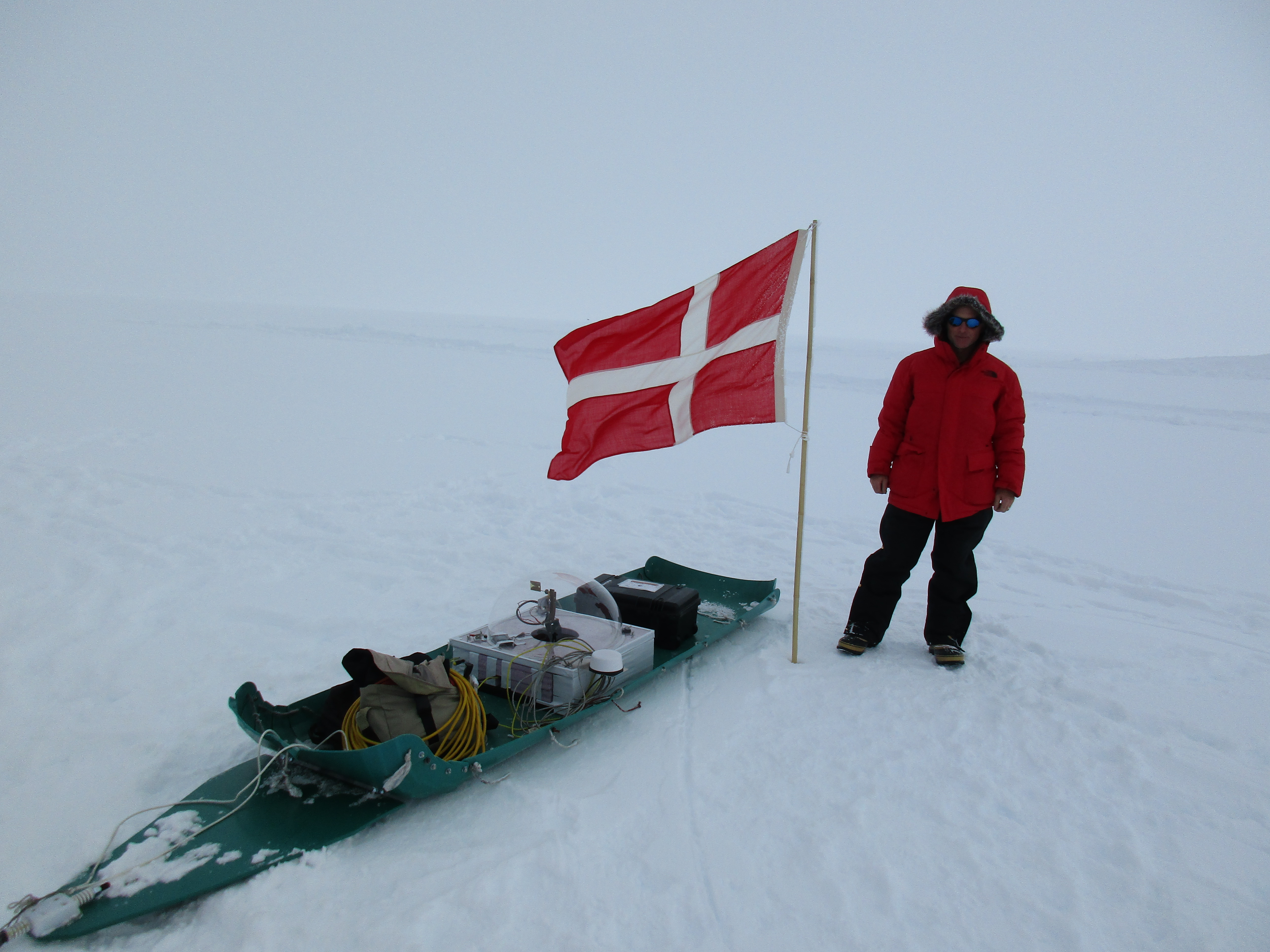
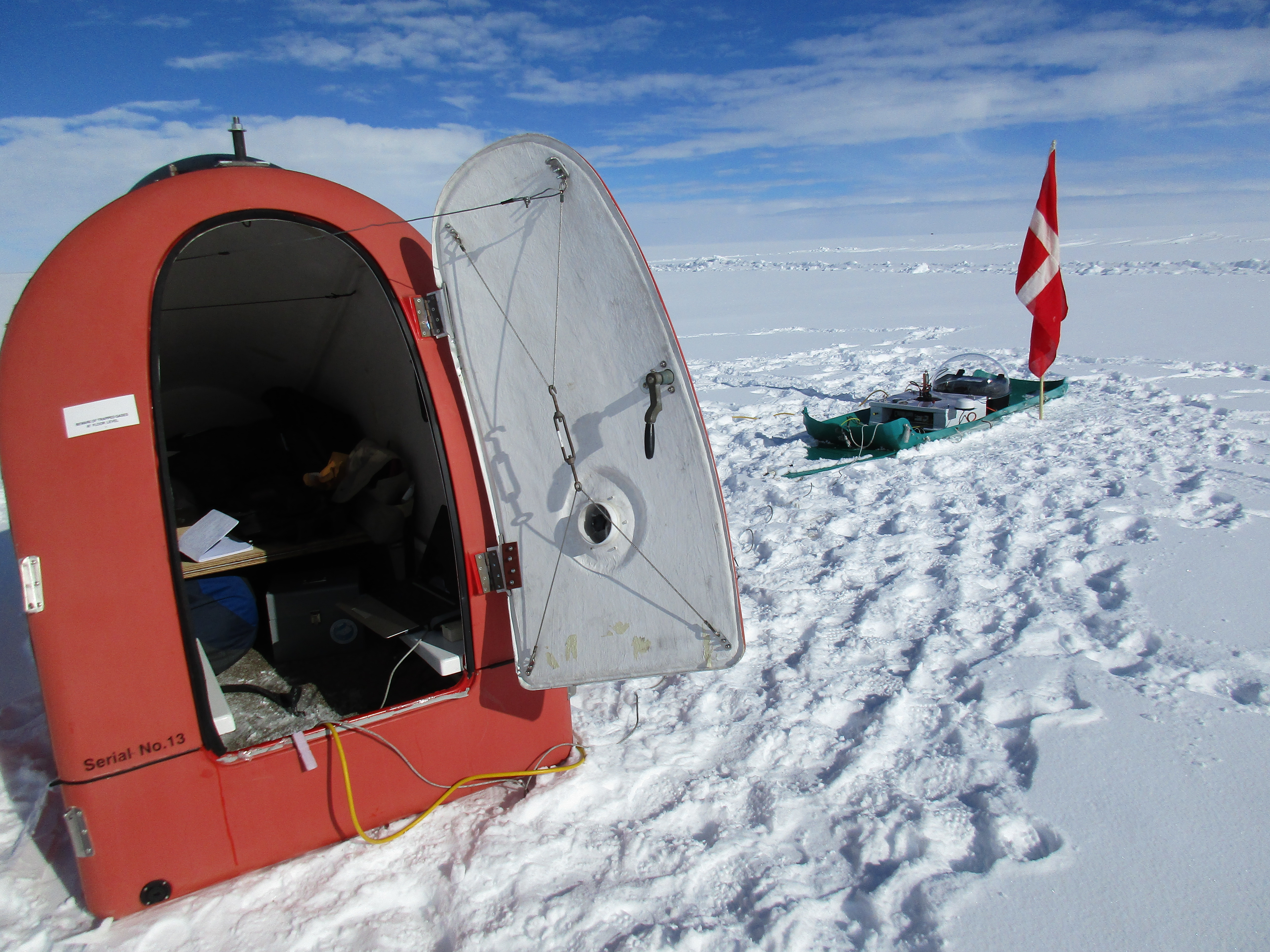
Workstation at Observation site
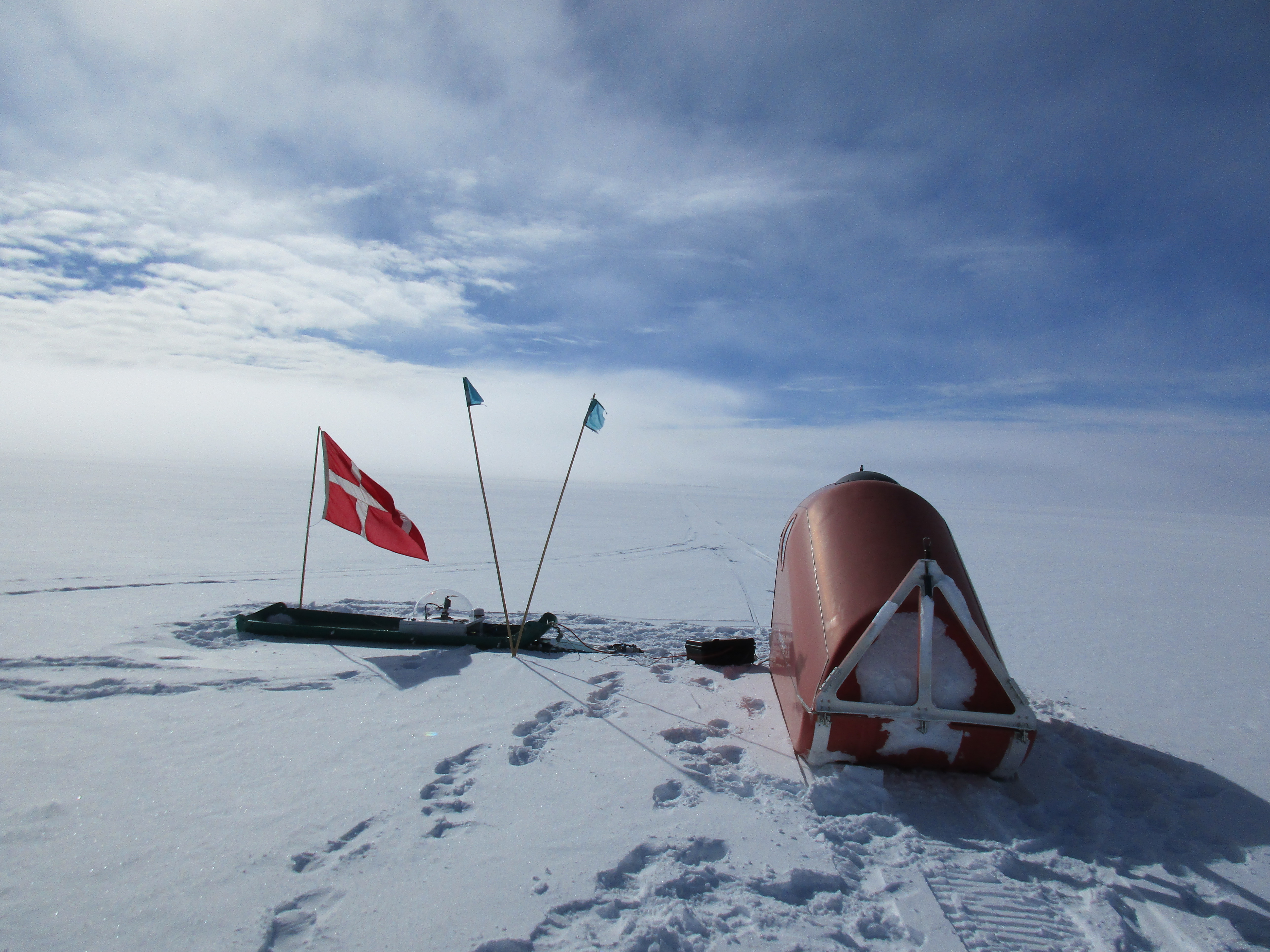
Greenland Scenery
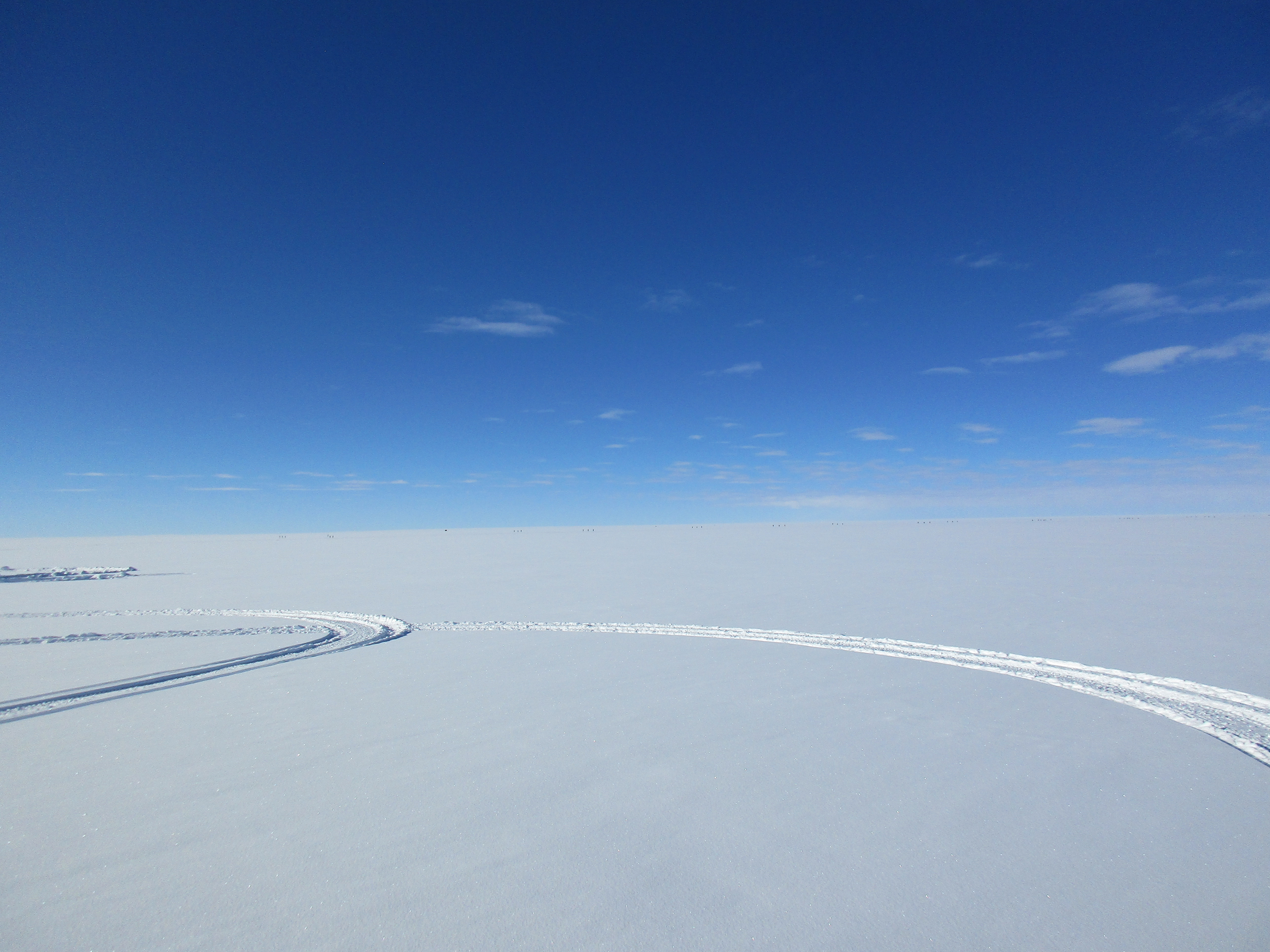
Greenland Scenery
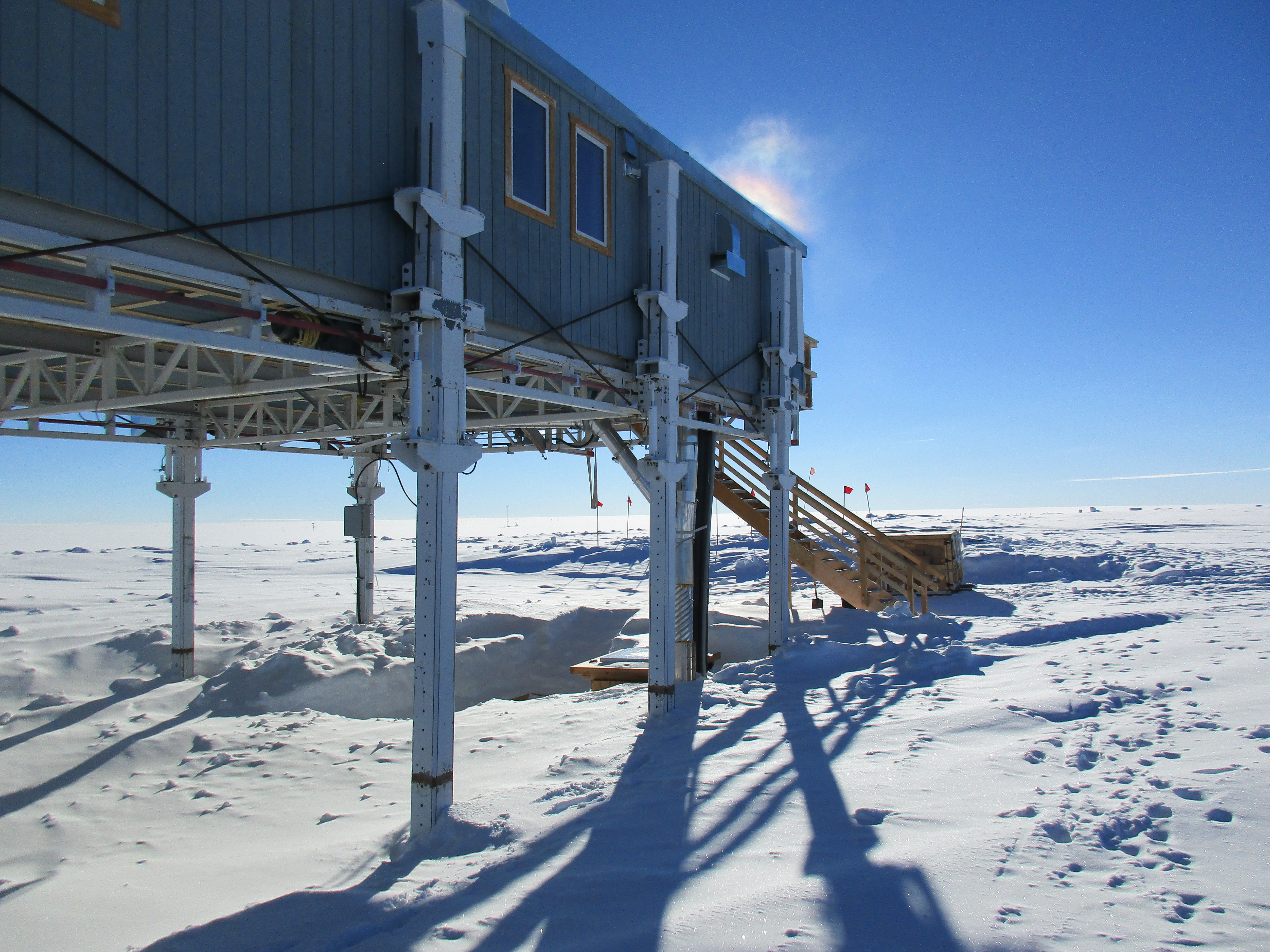
Adjusting for snow levels
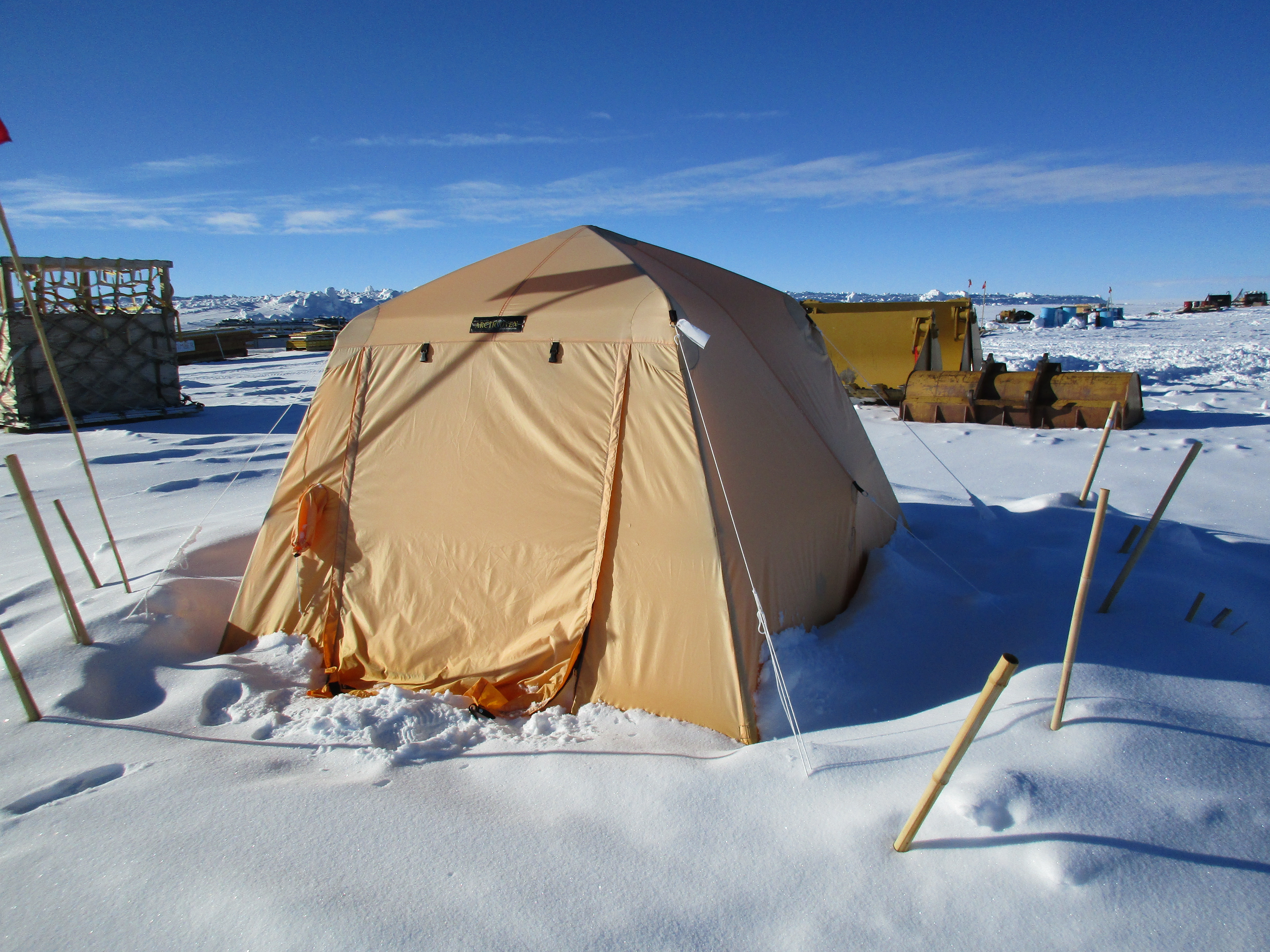
Sleeping accomodations
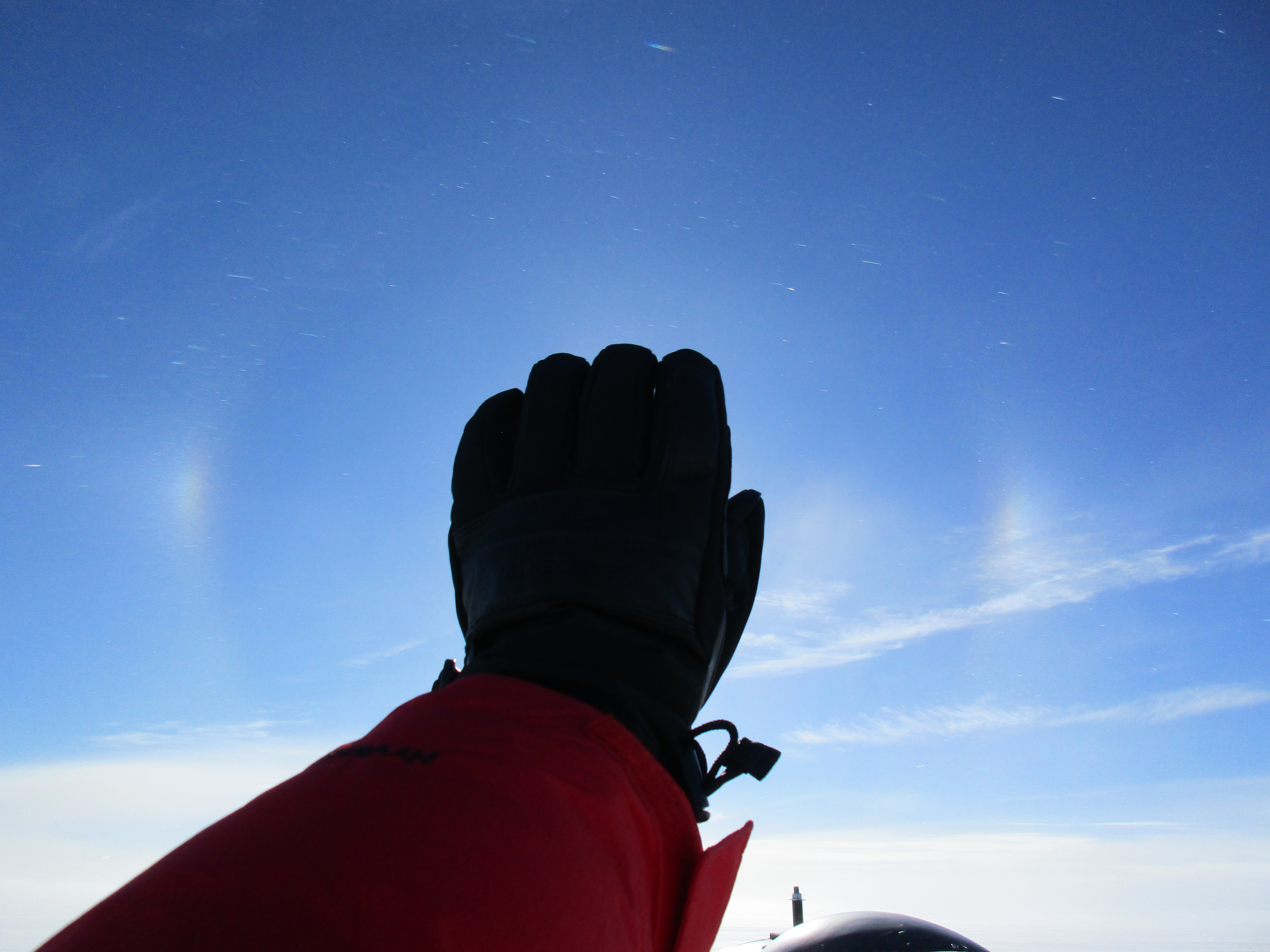
Sun Dogs
Greenland Deployment Summer 2018:
We successfully deployed and field tested our GreenPol system to Summit Station, Greenland from June 29 to August 13. While weather conditions presented more challenges than anticipated, we were able verify the functionality of our instrument design and shipping methods and are now able to provide a detailed account of observing conditions and infrastructure for the summer season. Our main take-away is that while cosmological or astrophysical studies from Summit Station are not impossible, there are significant obstacles to overcome based on our experience. The telescope was successfully shipped back to Santa Barbara on October 8th 2018 and stored at a location near campus. We are currently set up to run where we are and are using the opportunity of a nearby observation site to improve upon the system and obtain additional data. Eventually we would like to move the instrument to our White Mountain observation site or another California based site for improved observing conditions.
White Mountain Research Station site survey: http://128.111.23.62/wordpress/wp-content/uploads/2010/08/2005_wmrs_newastronomy.pdf
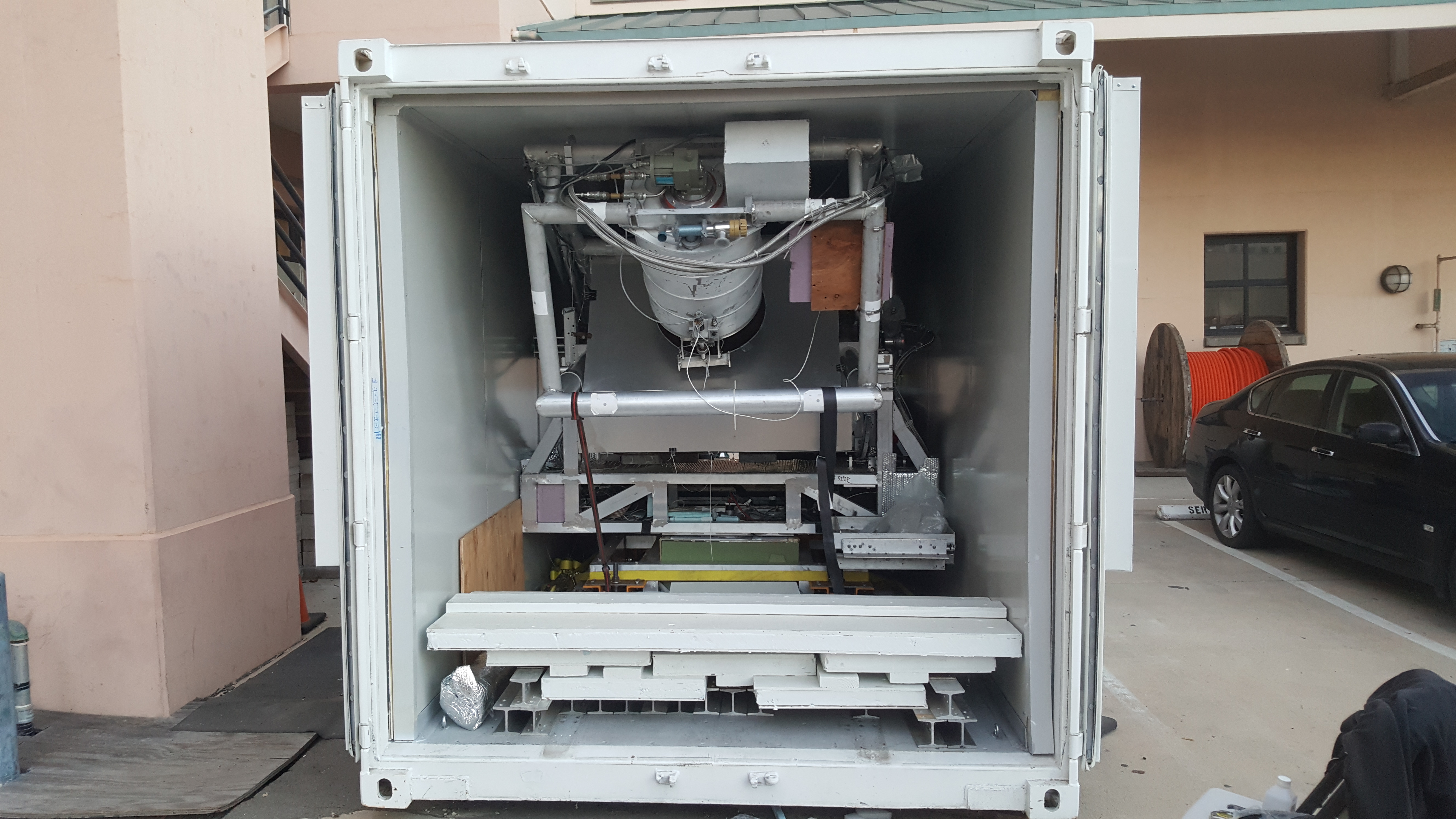
Everything we need got shipped in the container
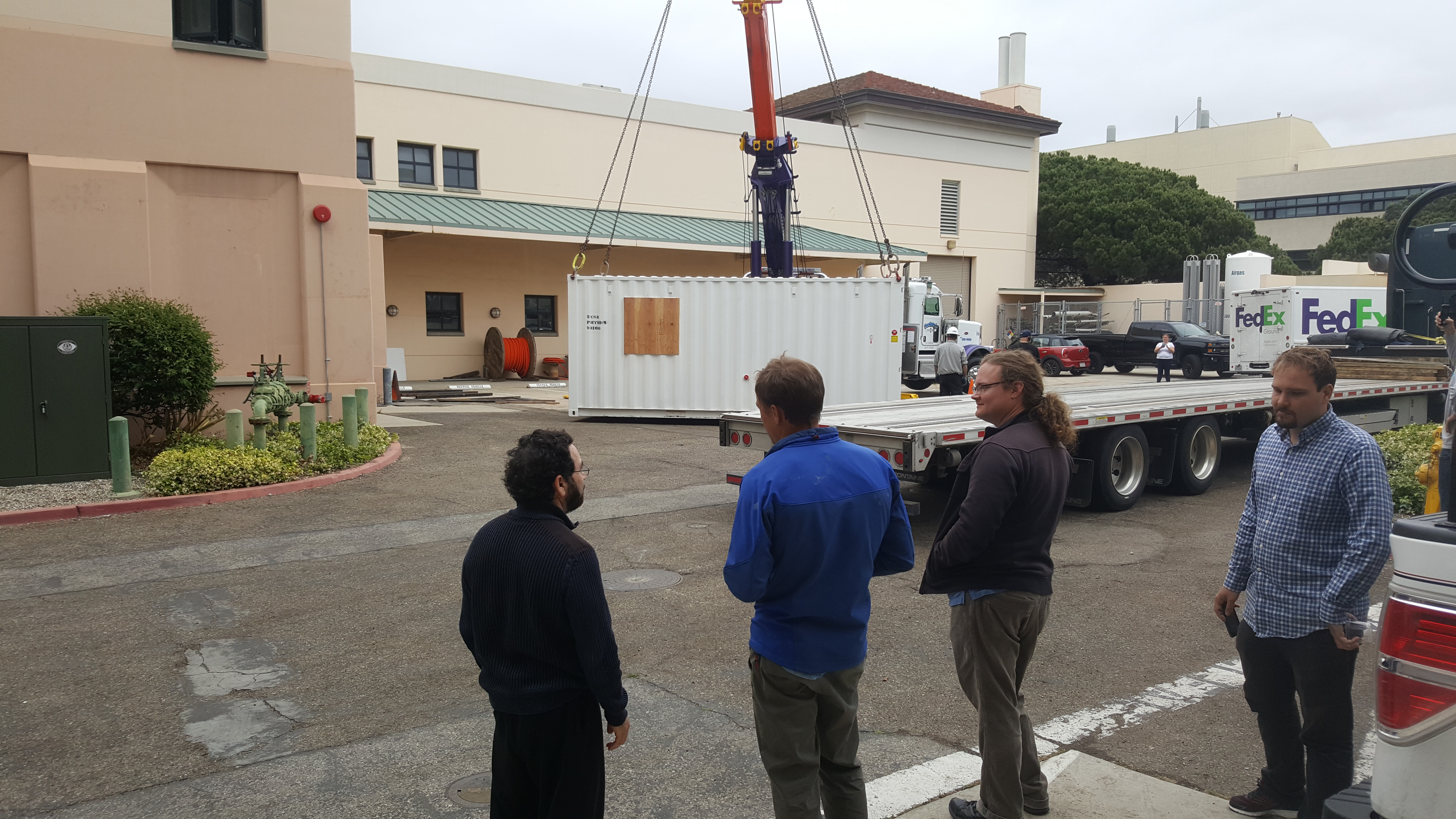
Telescope getting ready to leave
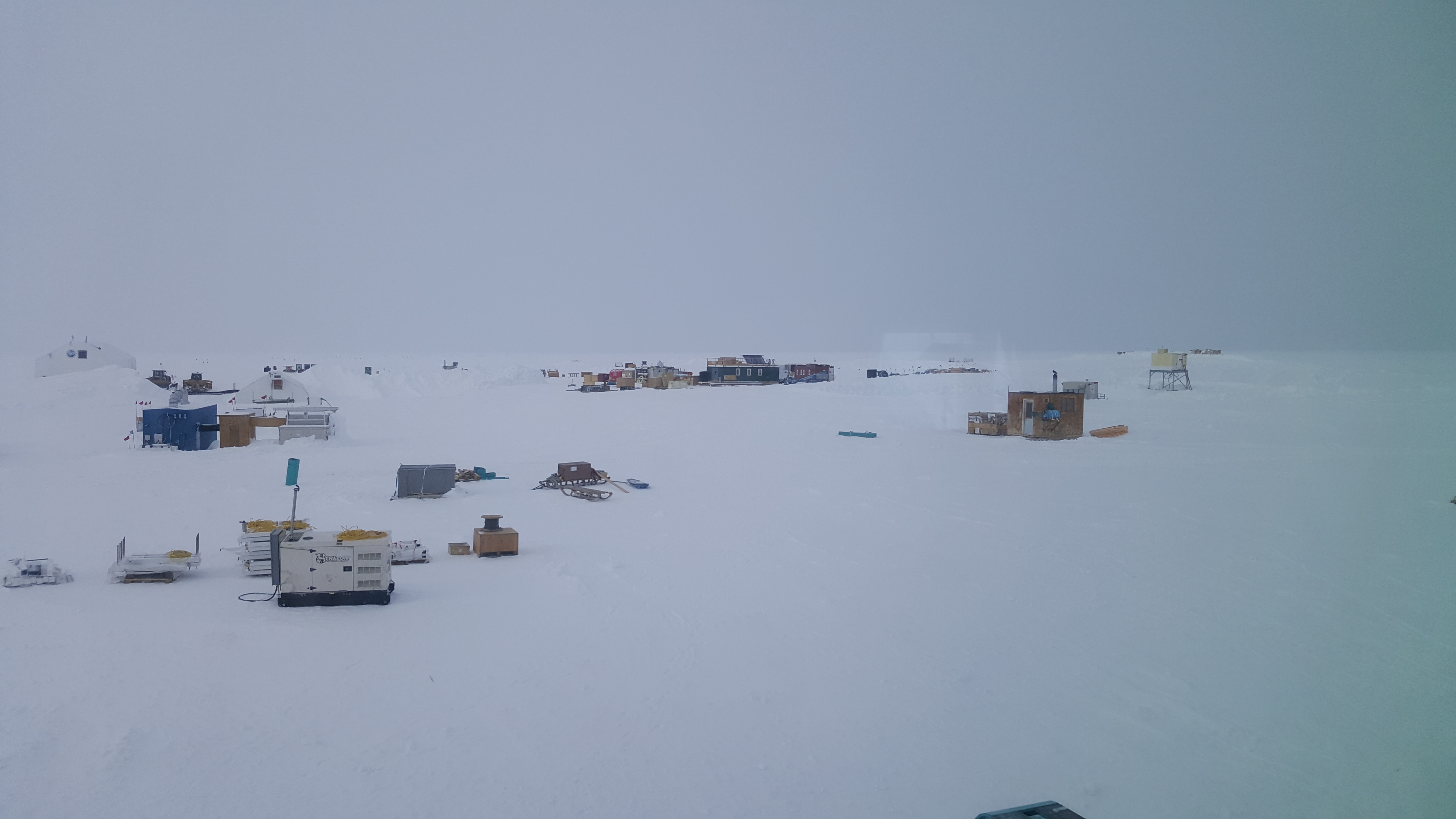
Summit Station in all its glory
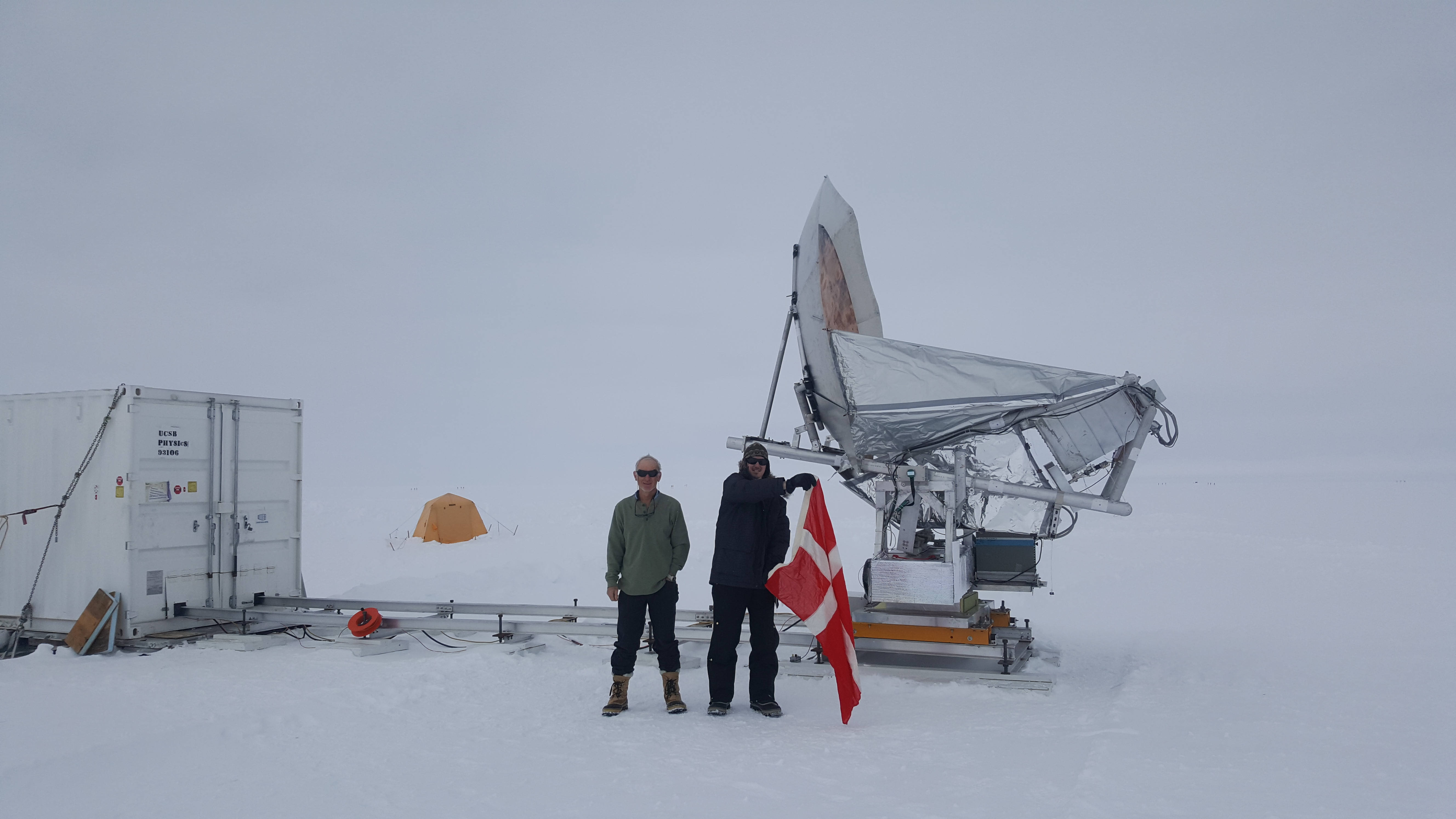
Repping Denmark
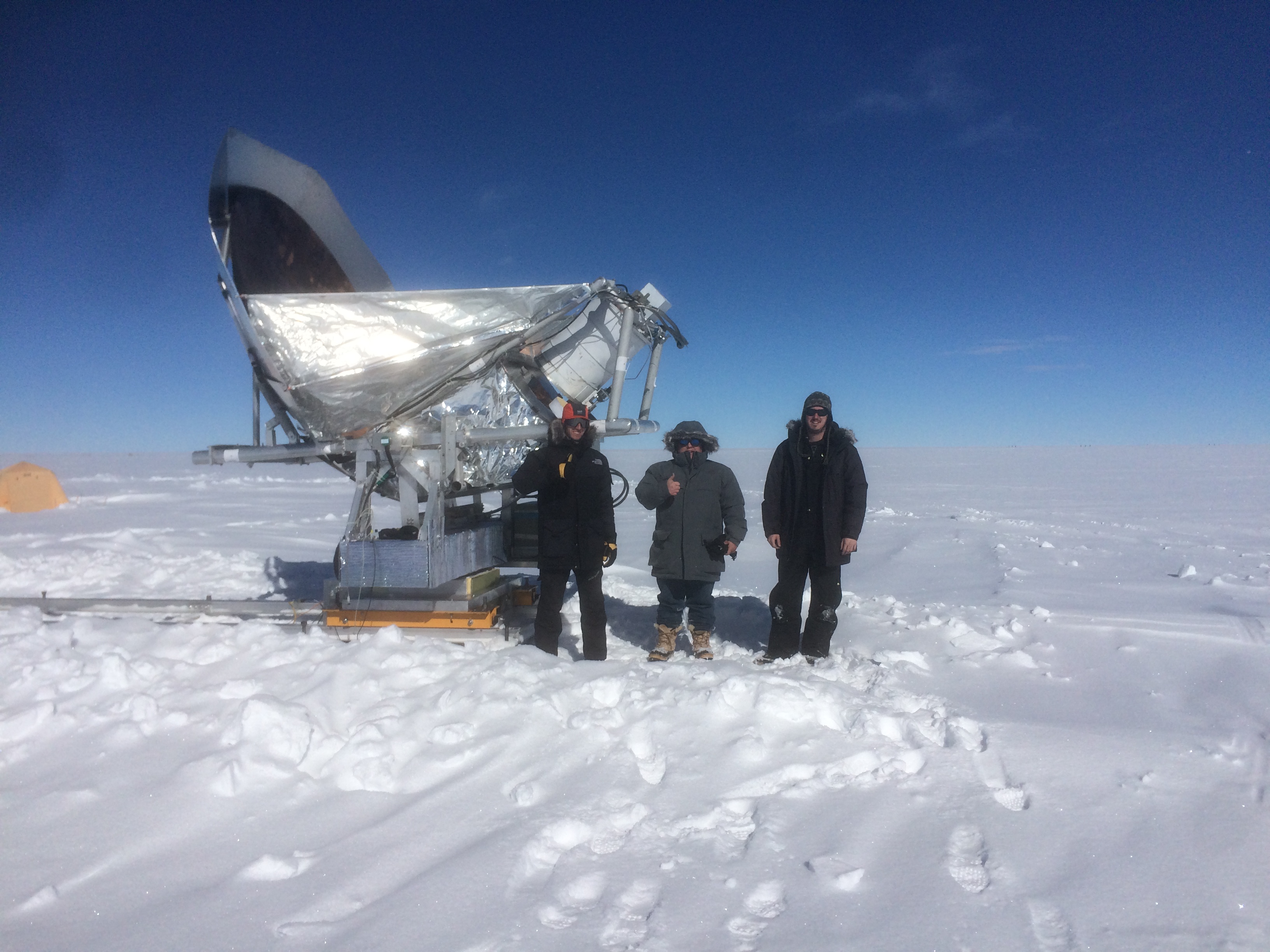
The Team: Harald Thommesen, Nic Rupert, Ari Kaplan
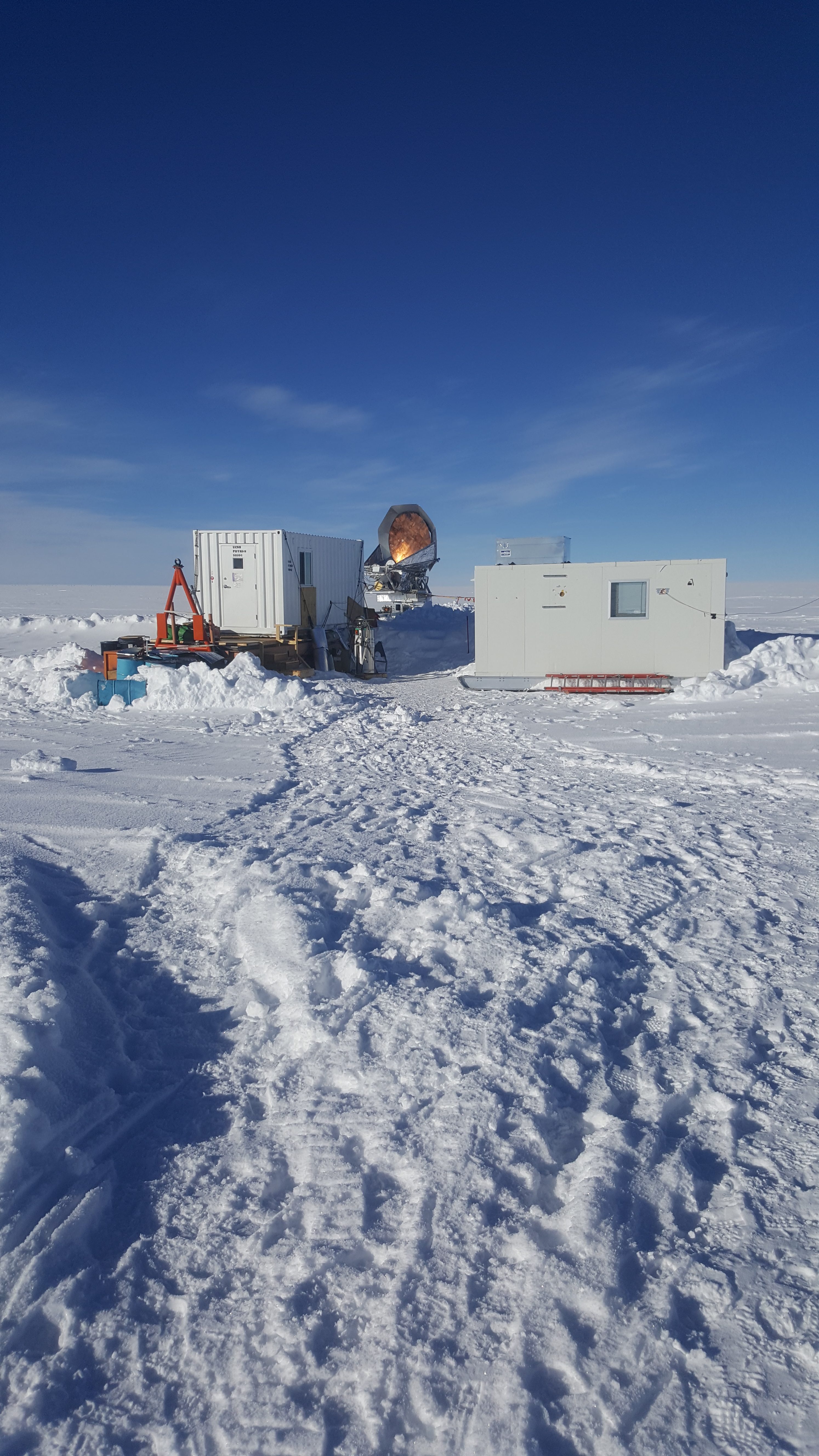
Work Station/My Apartment
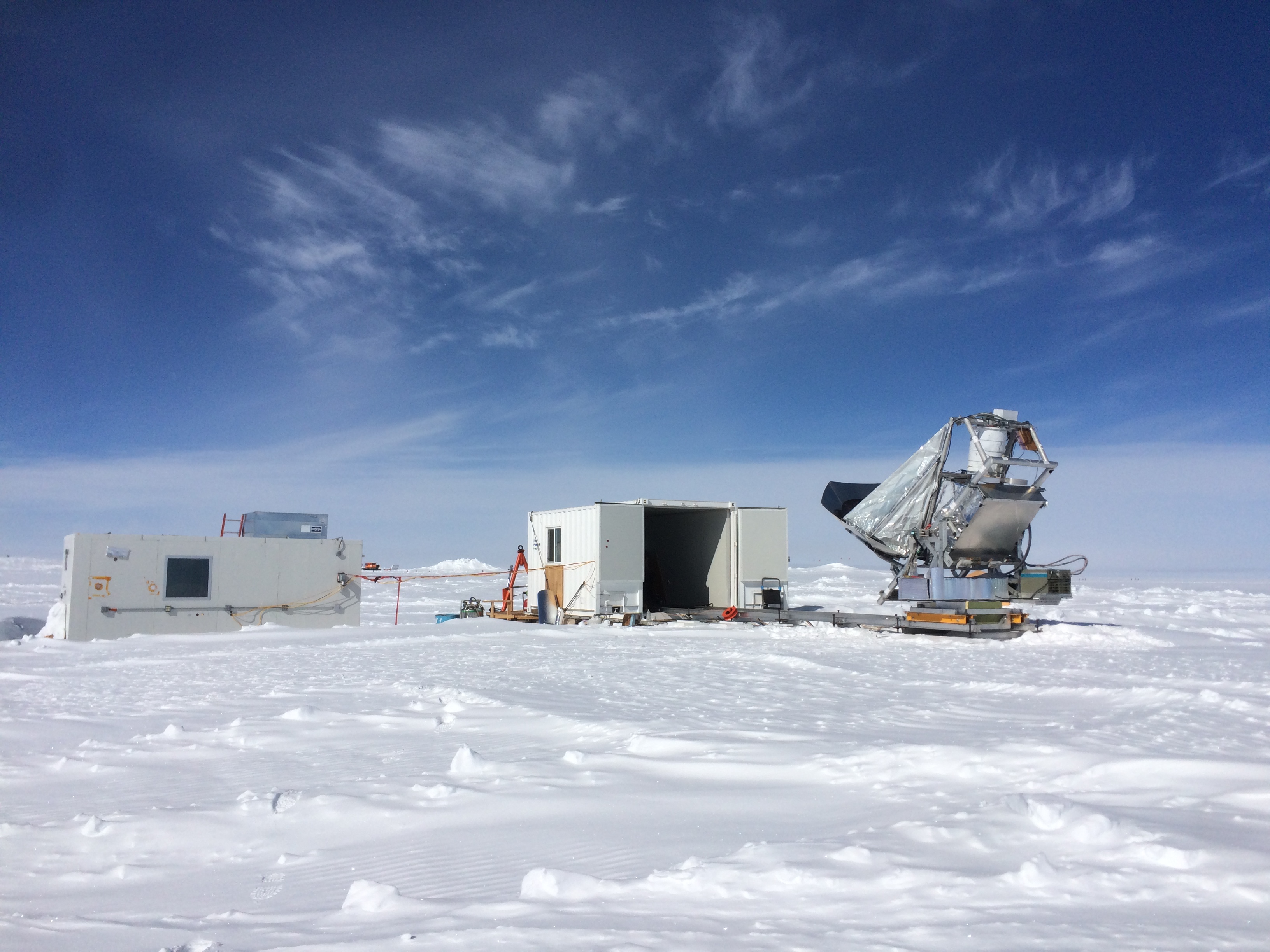
Fully Deployed System
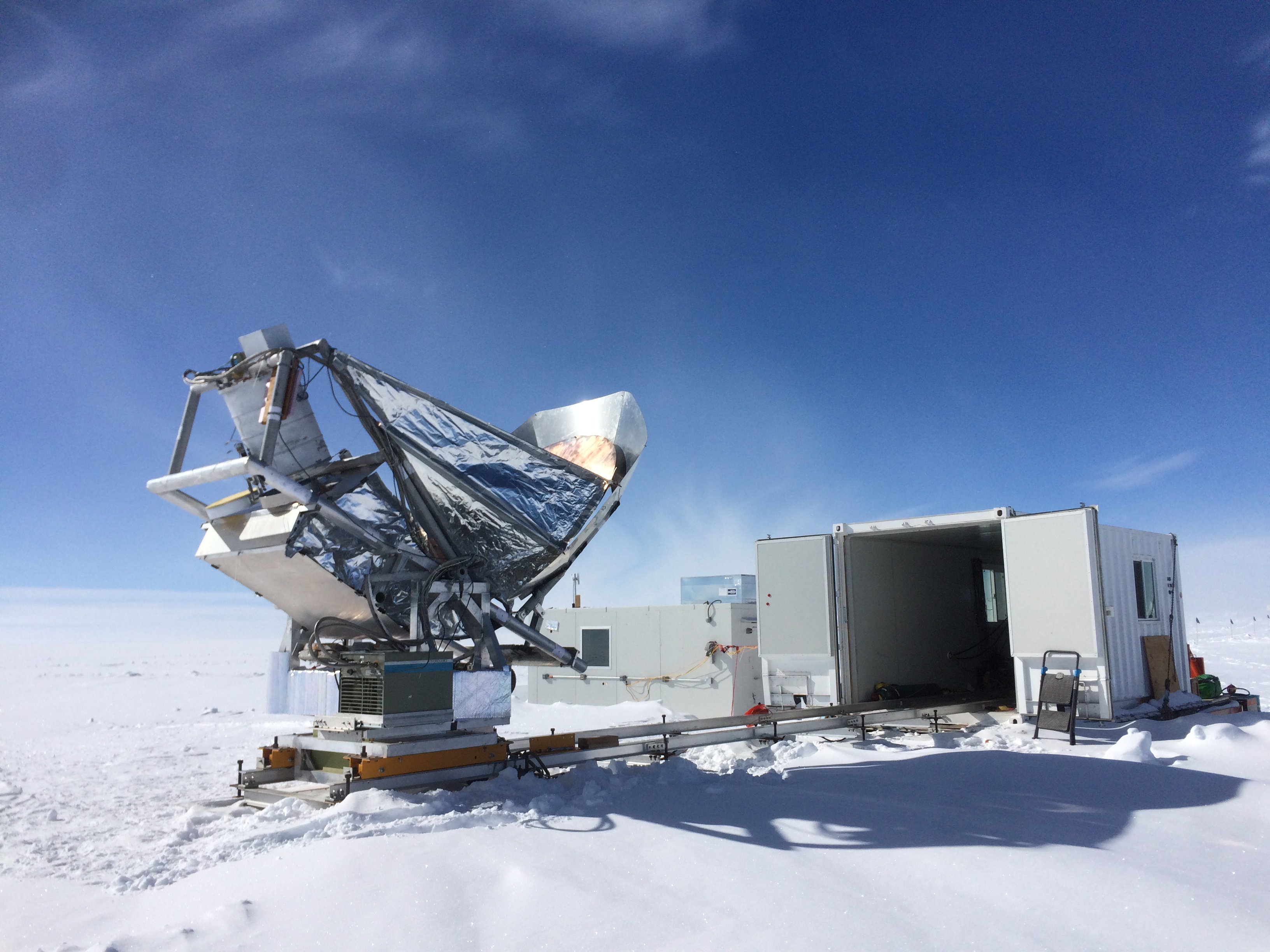
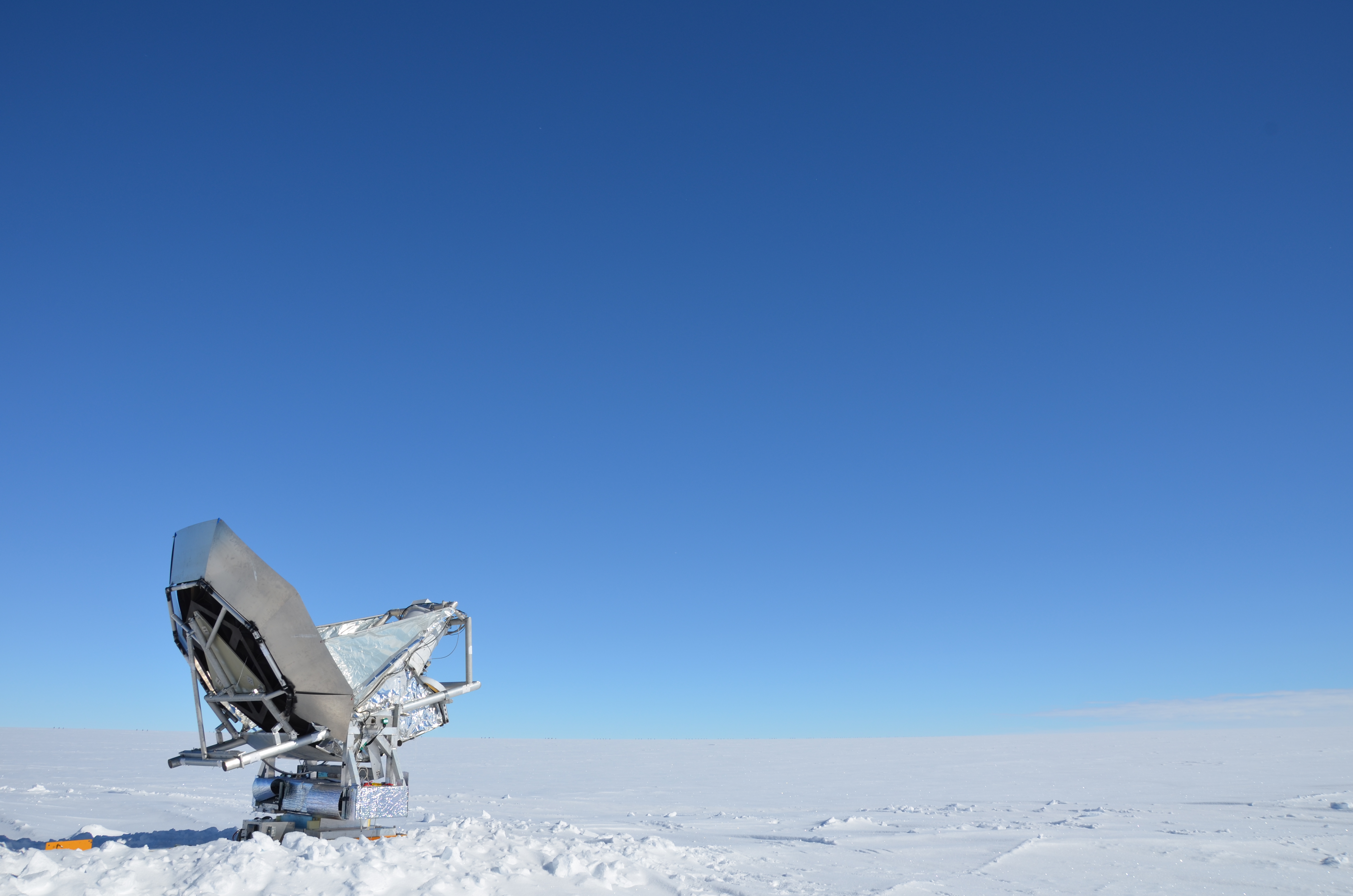
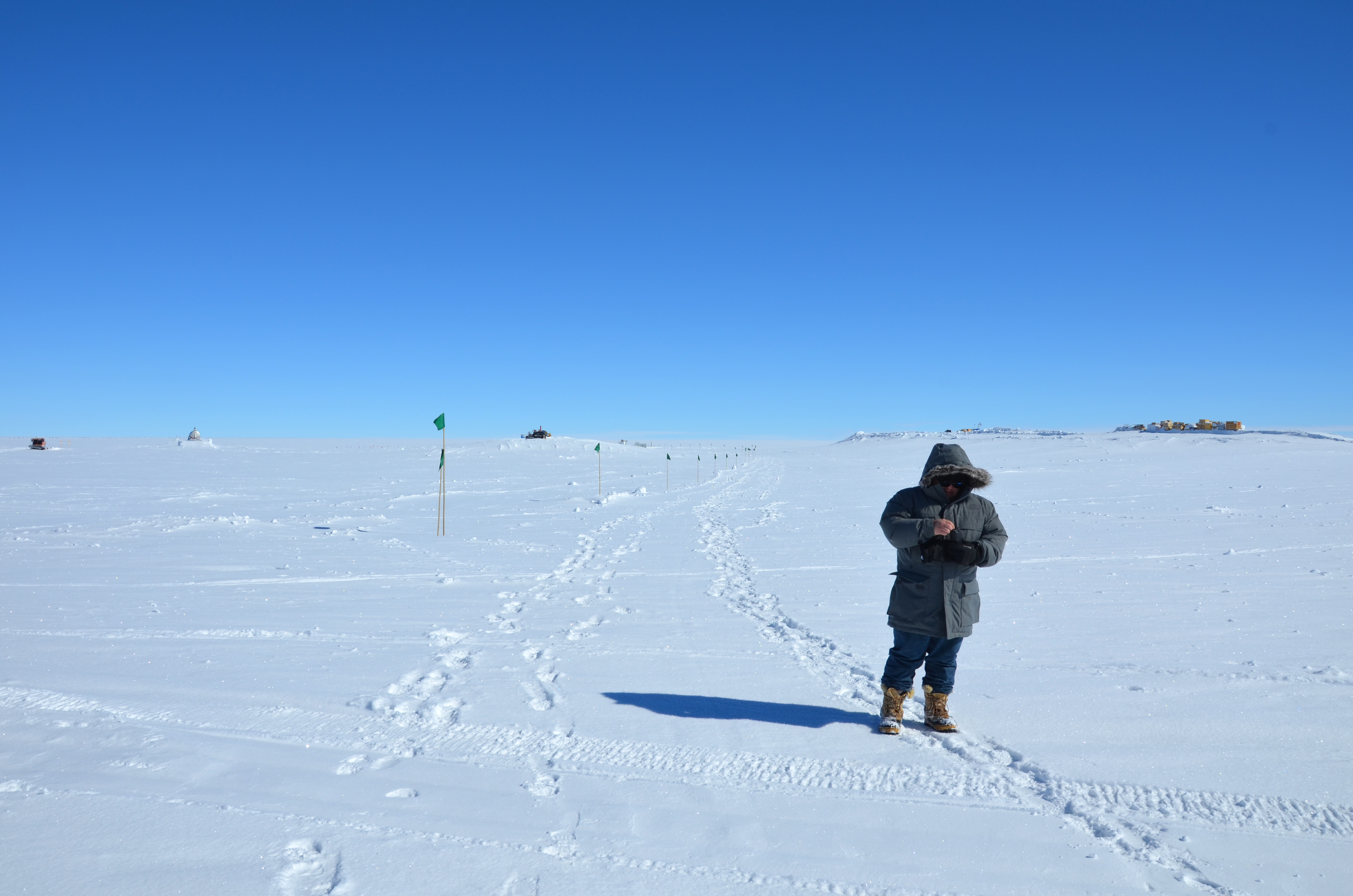
That's Nic
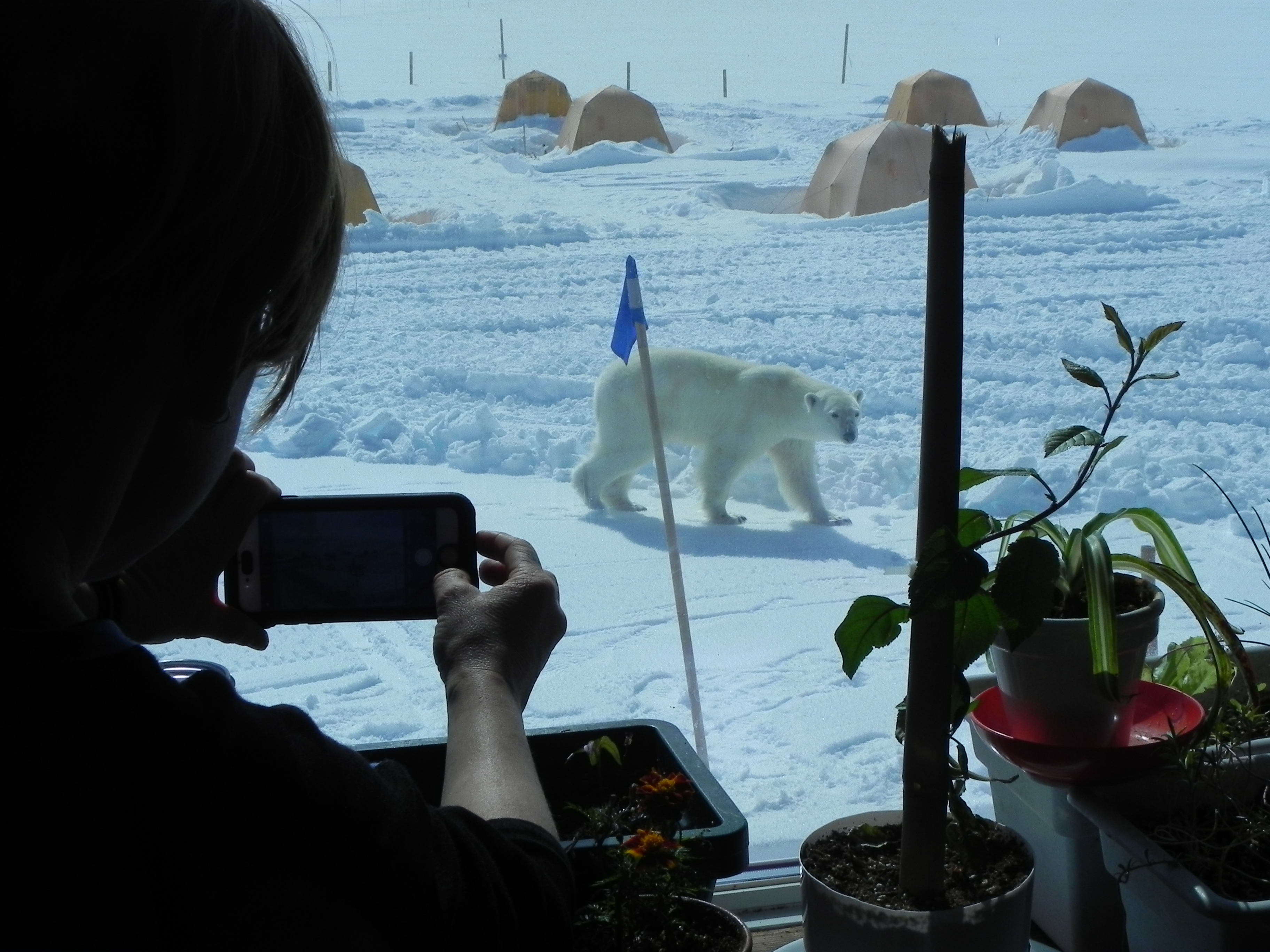
Team mascot (2 weeks before we got there)
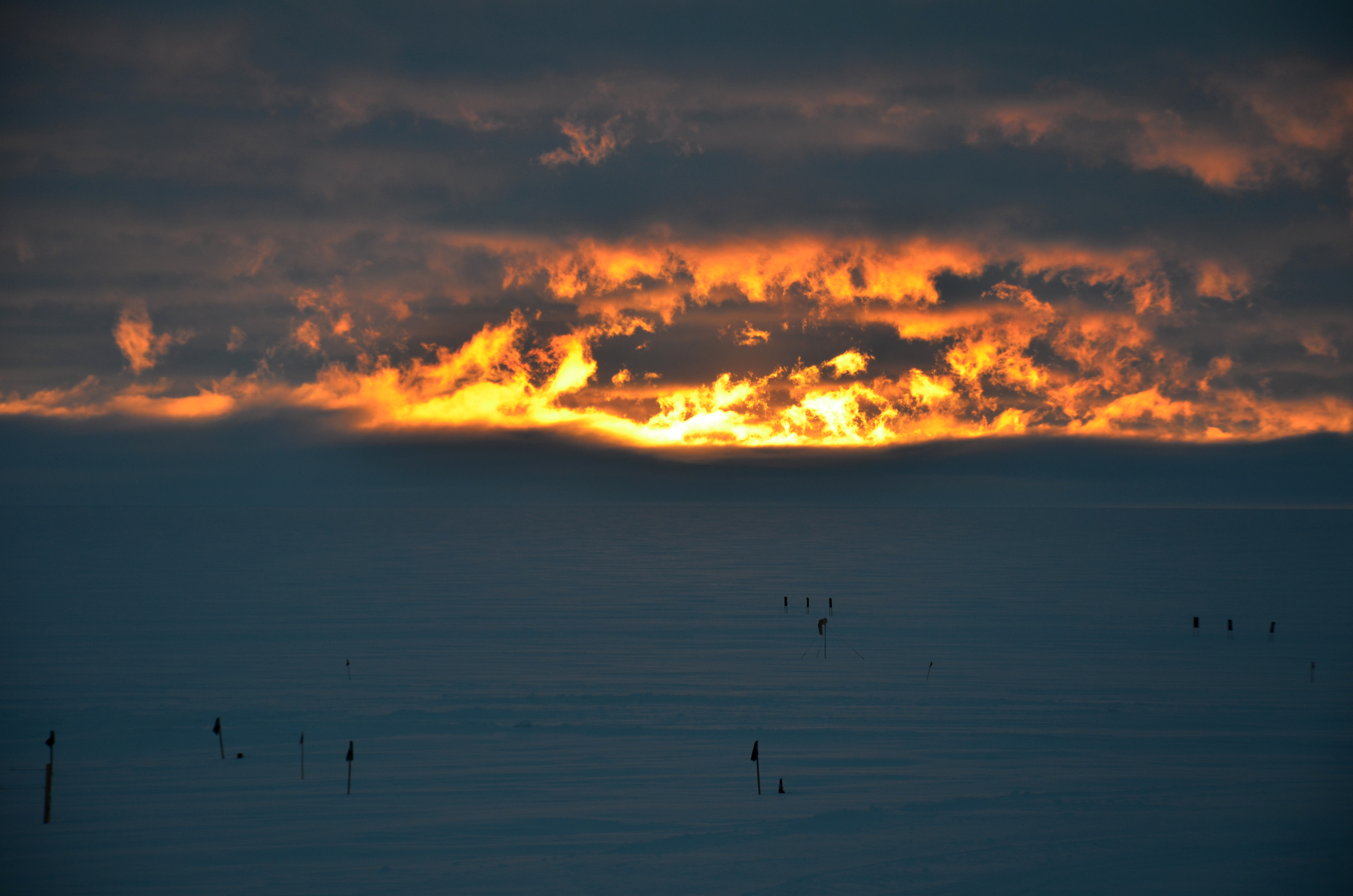
First sunset of the year
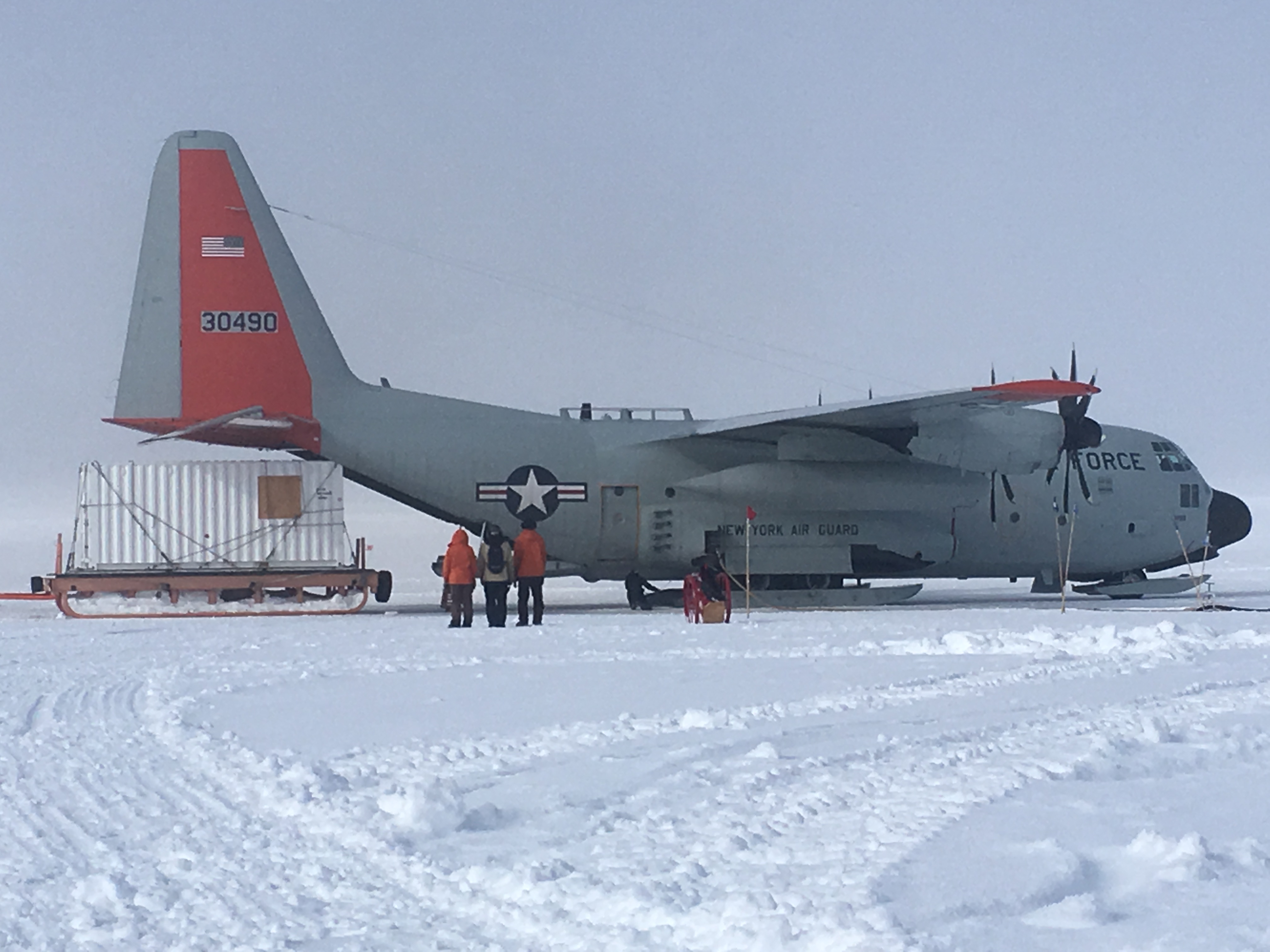
Loading up to go home on C-130
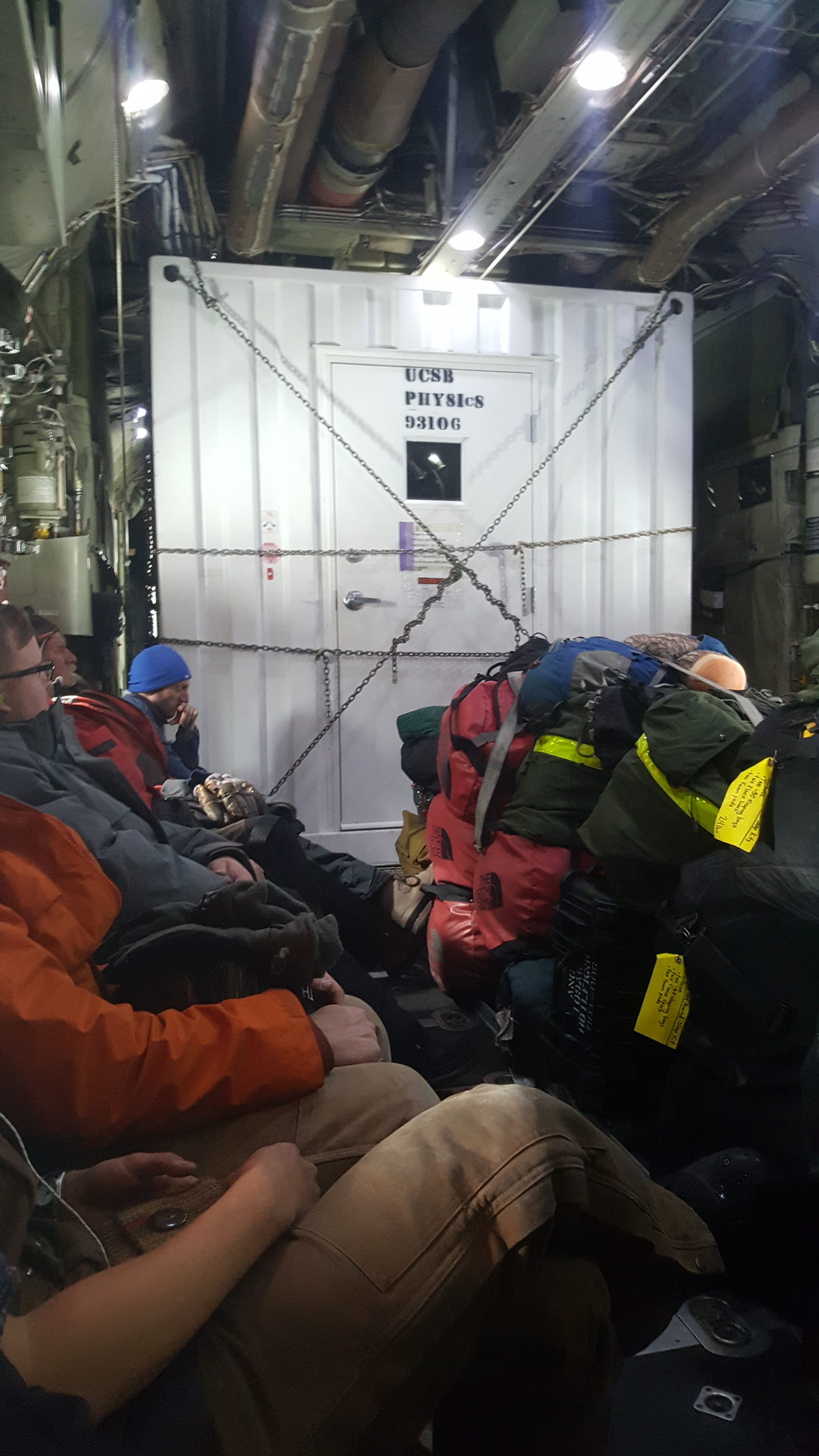
Look it's Nic again.
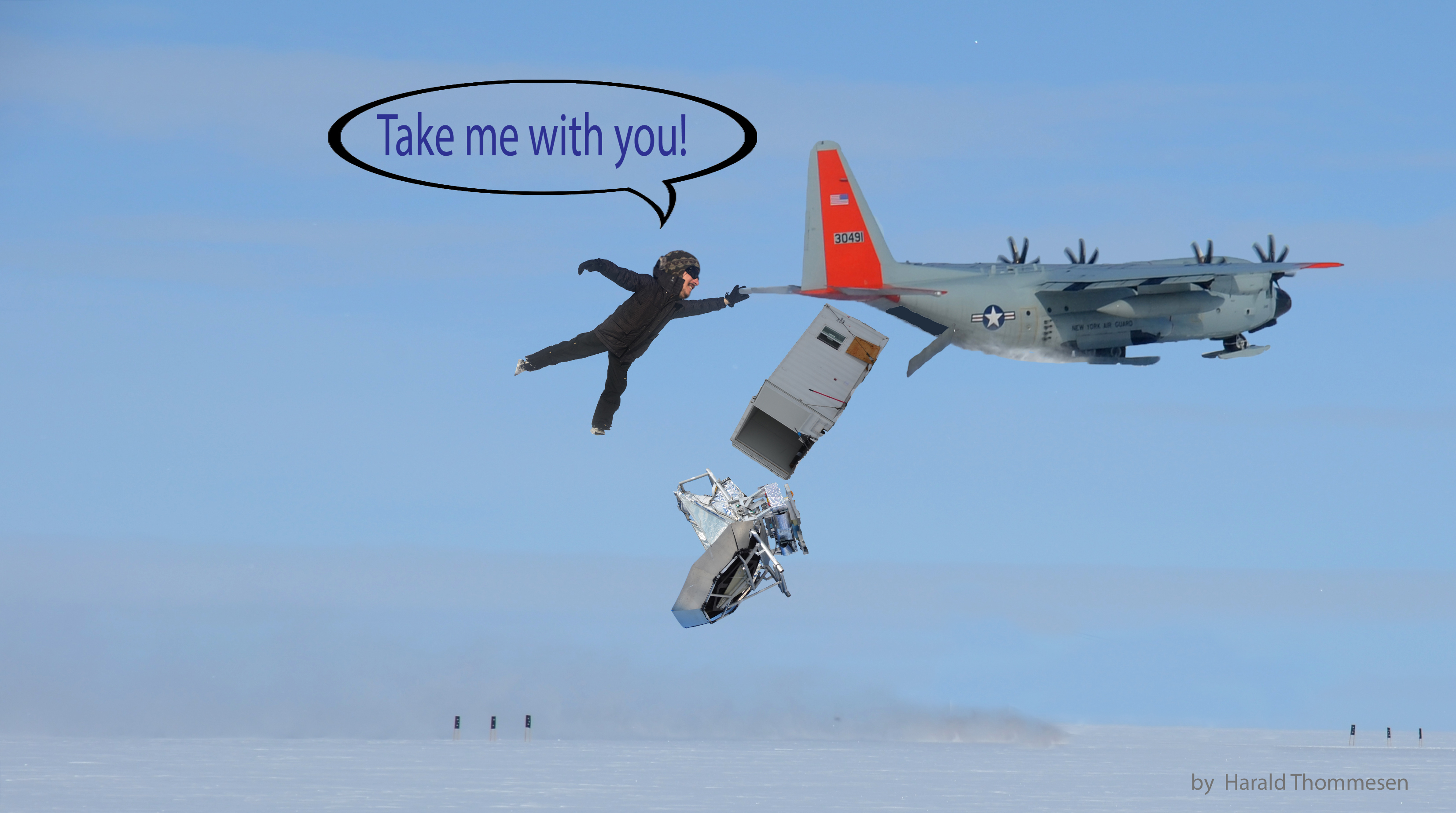
This is exactly how it happened.
Components of GreenPol:
Due to the variable weather at Summit, we need a system which is both rapidly deployable and retractable. In order to accomplish this we modified a standard 20 ft. ISO container with a 40 ft. track system which can slide the telescope into and out of the container when needed. The optical frame rests on a mechanical sub-frame which houses the electronics, azimuth and elevation drive, and track sled. The optics include a primary mirror, grid polarizer, secondary mirror, and detector array. The grid polarizer is a spinning linear polarizing grid that modulates the U and Q polarizations of incoming photons, allowing us to measure them both simultaneously with low loss. This is important since the goal is to measure the microwave polarization. The detector arrays are made up of feed horns that guide the microwaves to antennas, which convert the waves into electrical signals. These signals are passed through low noise amplifiers that must be kept very cold (around 20 Kelvin) to minimize noise. To be able to cool the system the detector arrays are mounted in vacuum tight dewars that are cooled with liquid helium (about 4 Kelvin) supplied by the on board storage dewars. All data, both housekeeping and scientific, will be synchronously acquired by a custom made FPGA system which is under development. After initial capture, the data is passed to a host processor for further analysis and storage.
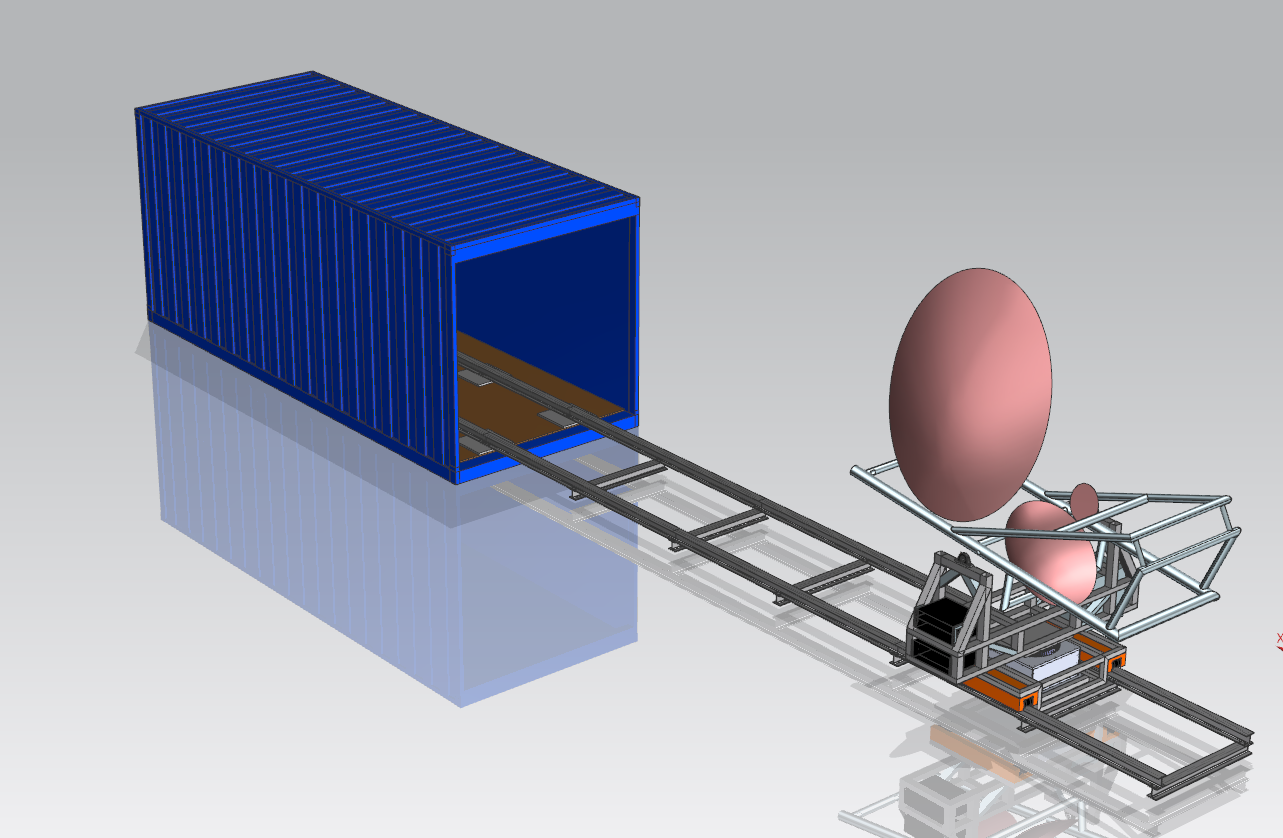
Full System Deployment
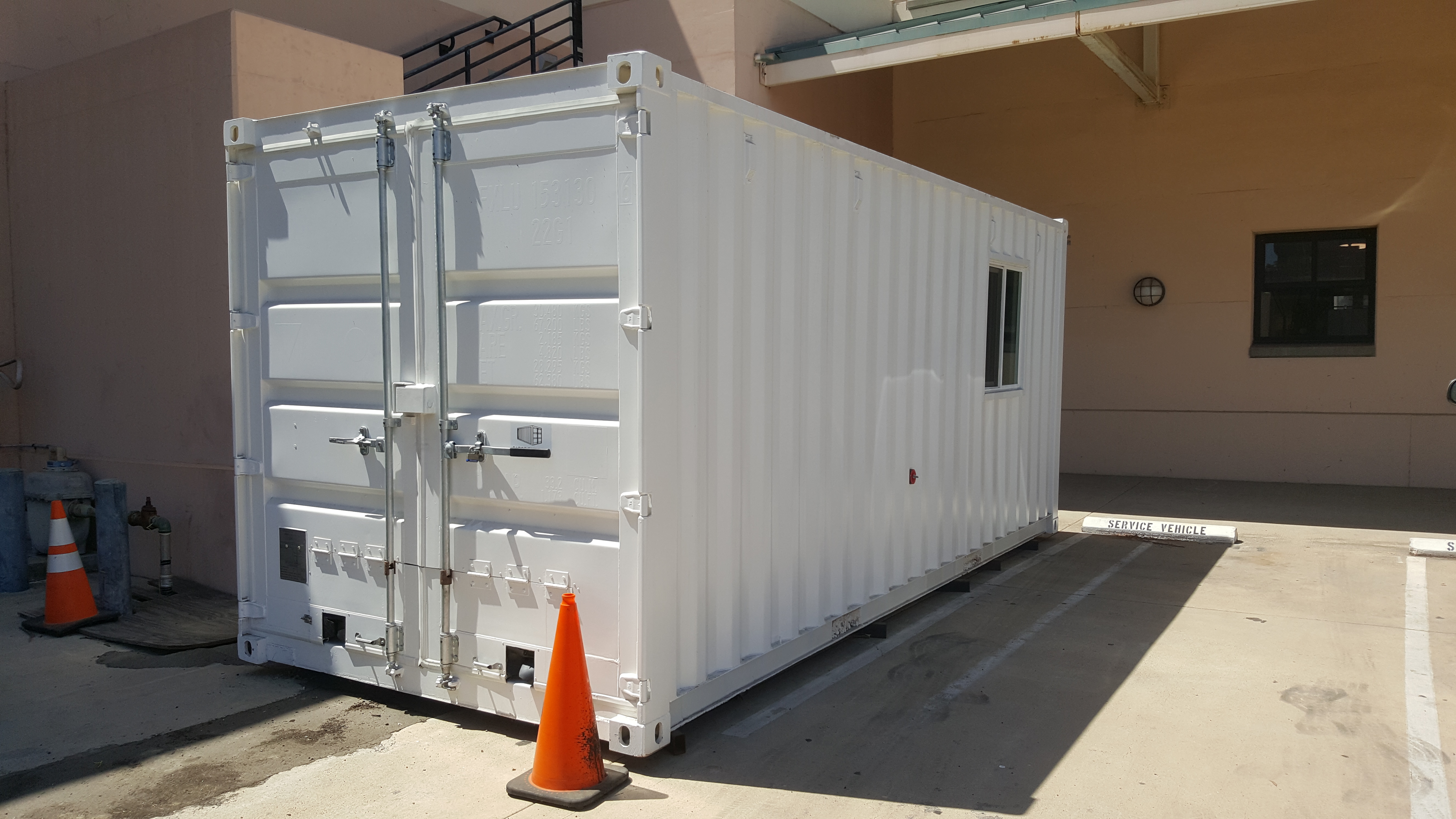
ISO container
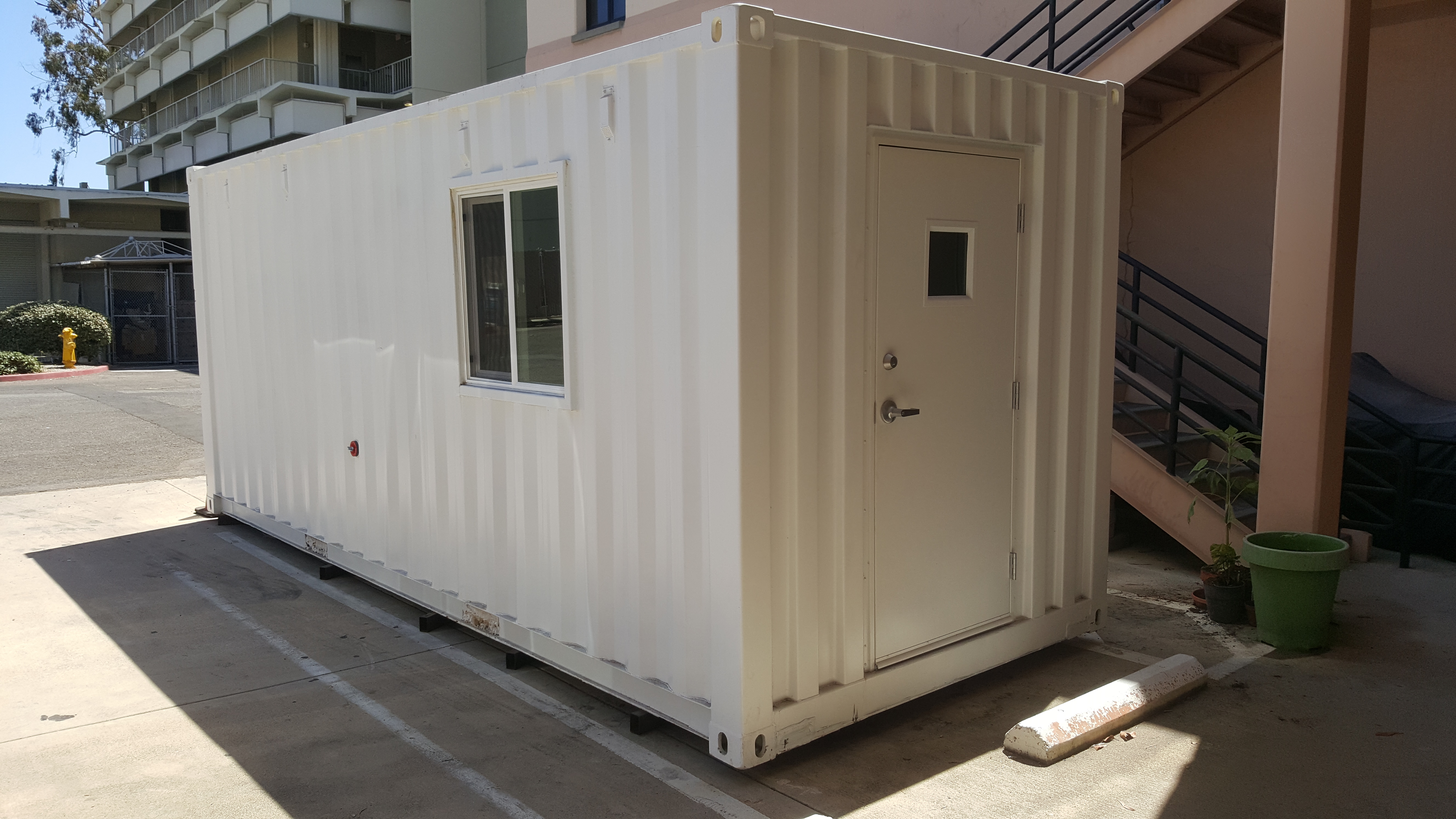
ISO container
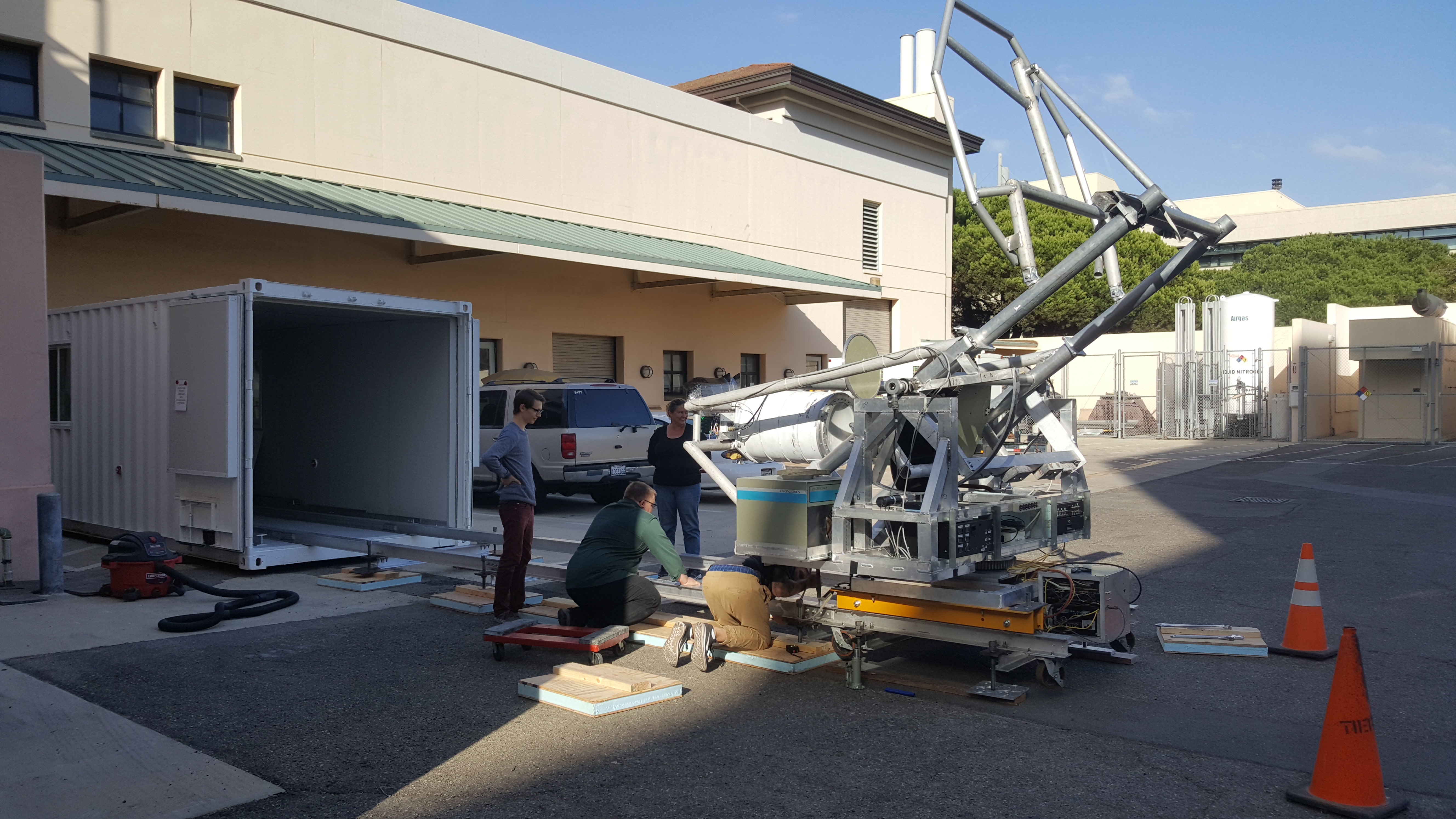
Deployment on track system
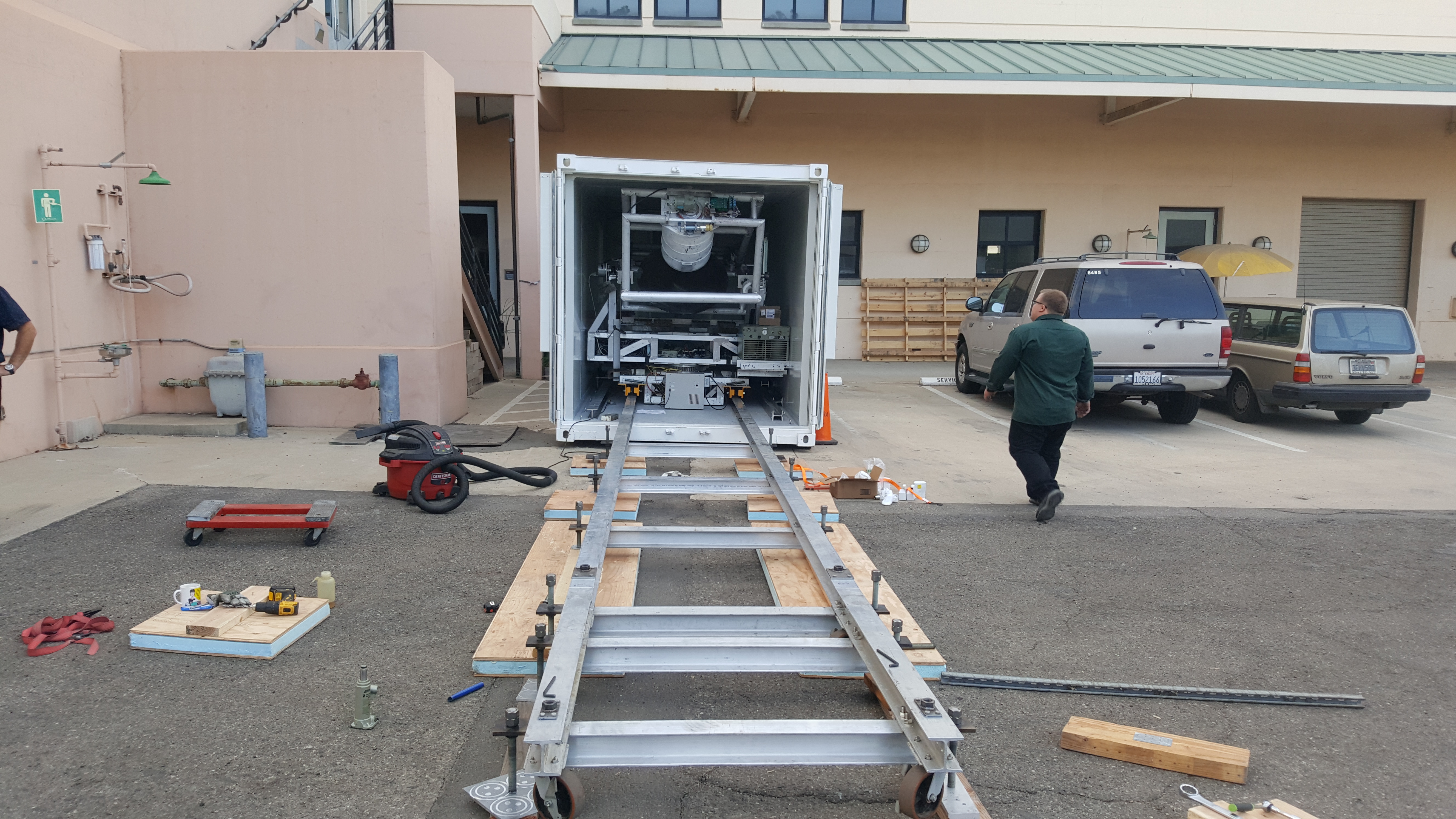
First install into container
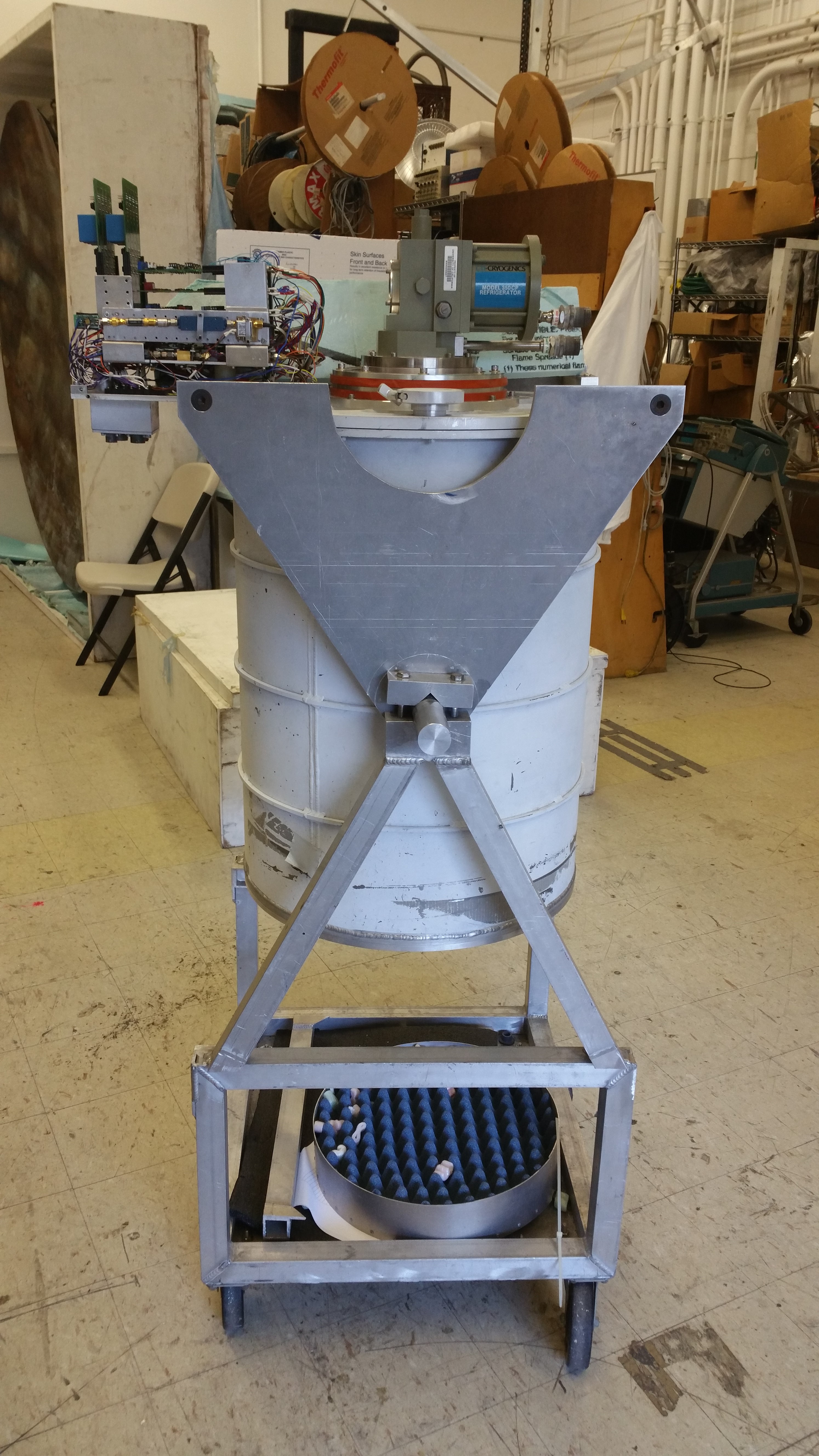
Detector Array
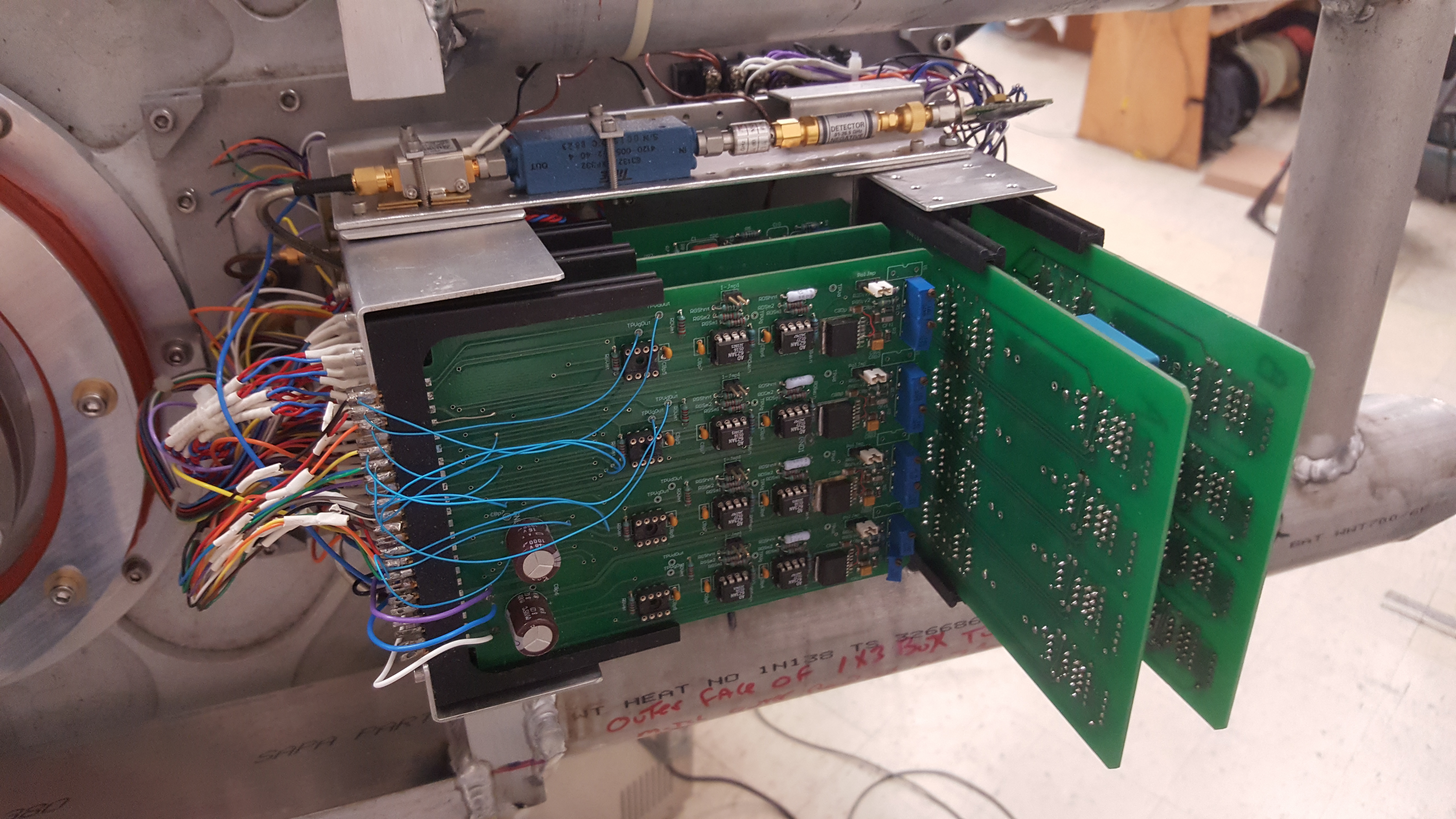
Detector Electronics
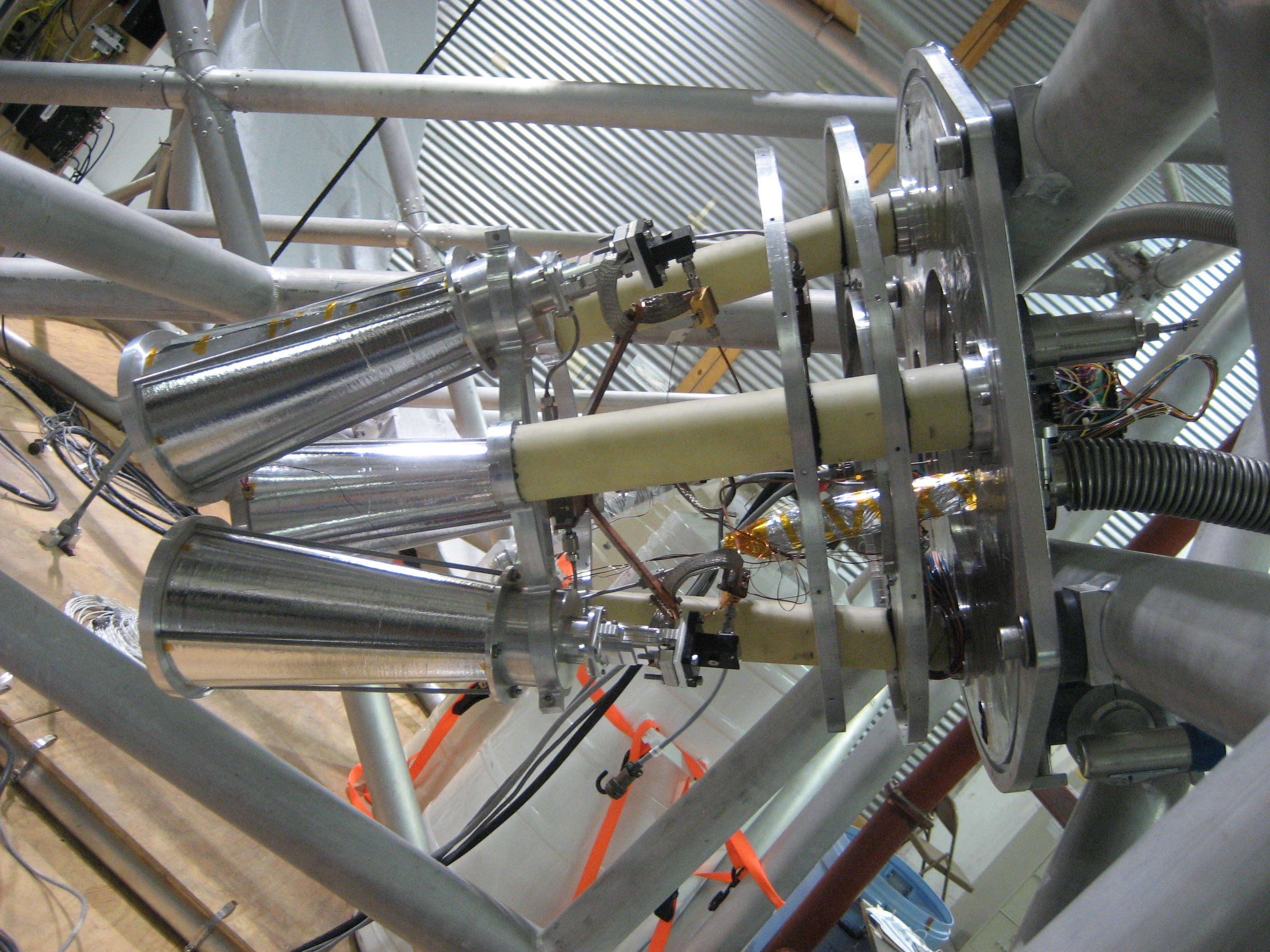
10 GHz Horns in Detector Array
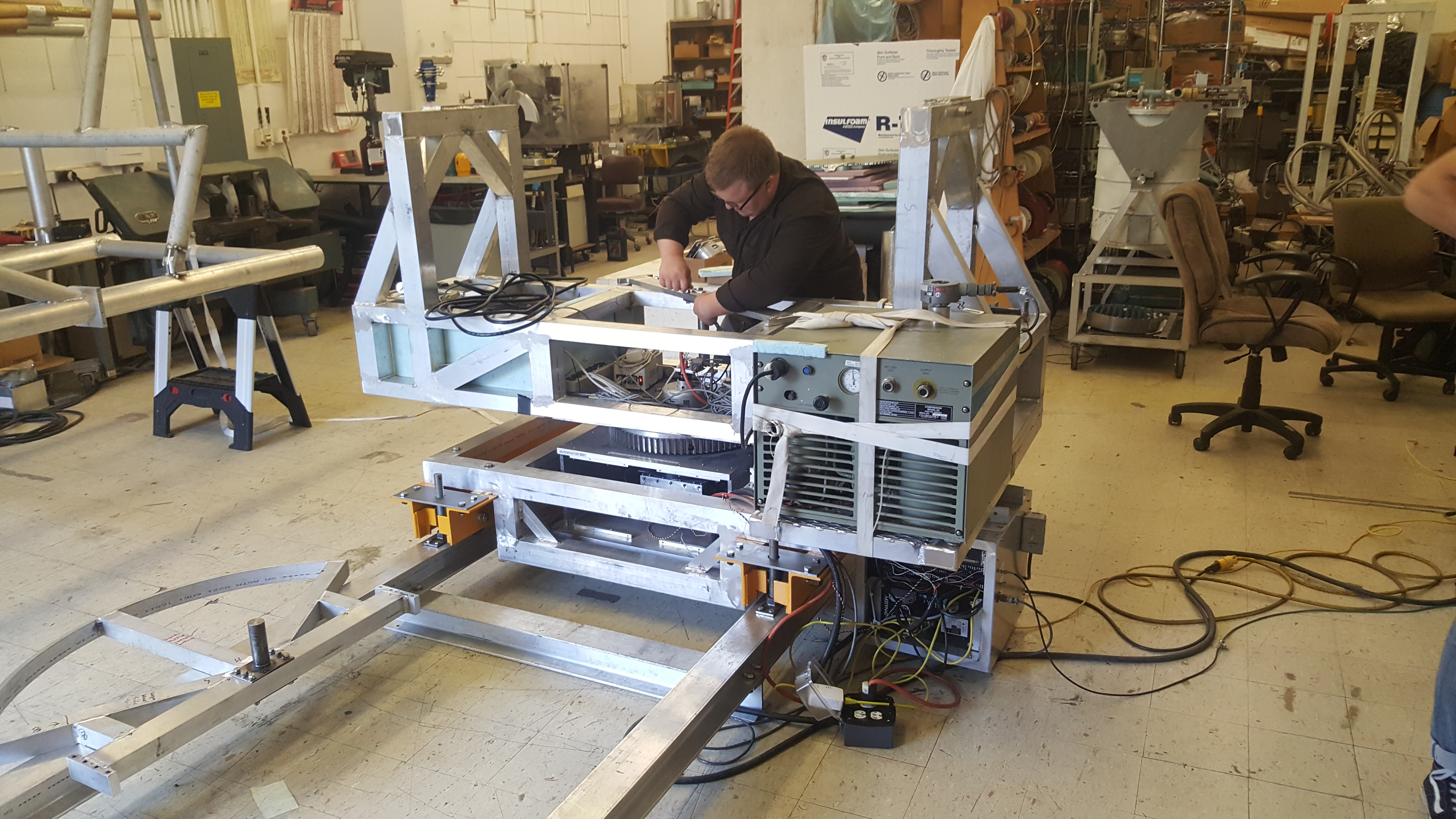
Mechanical Sub-Frame
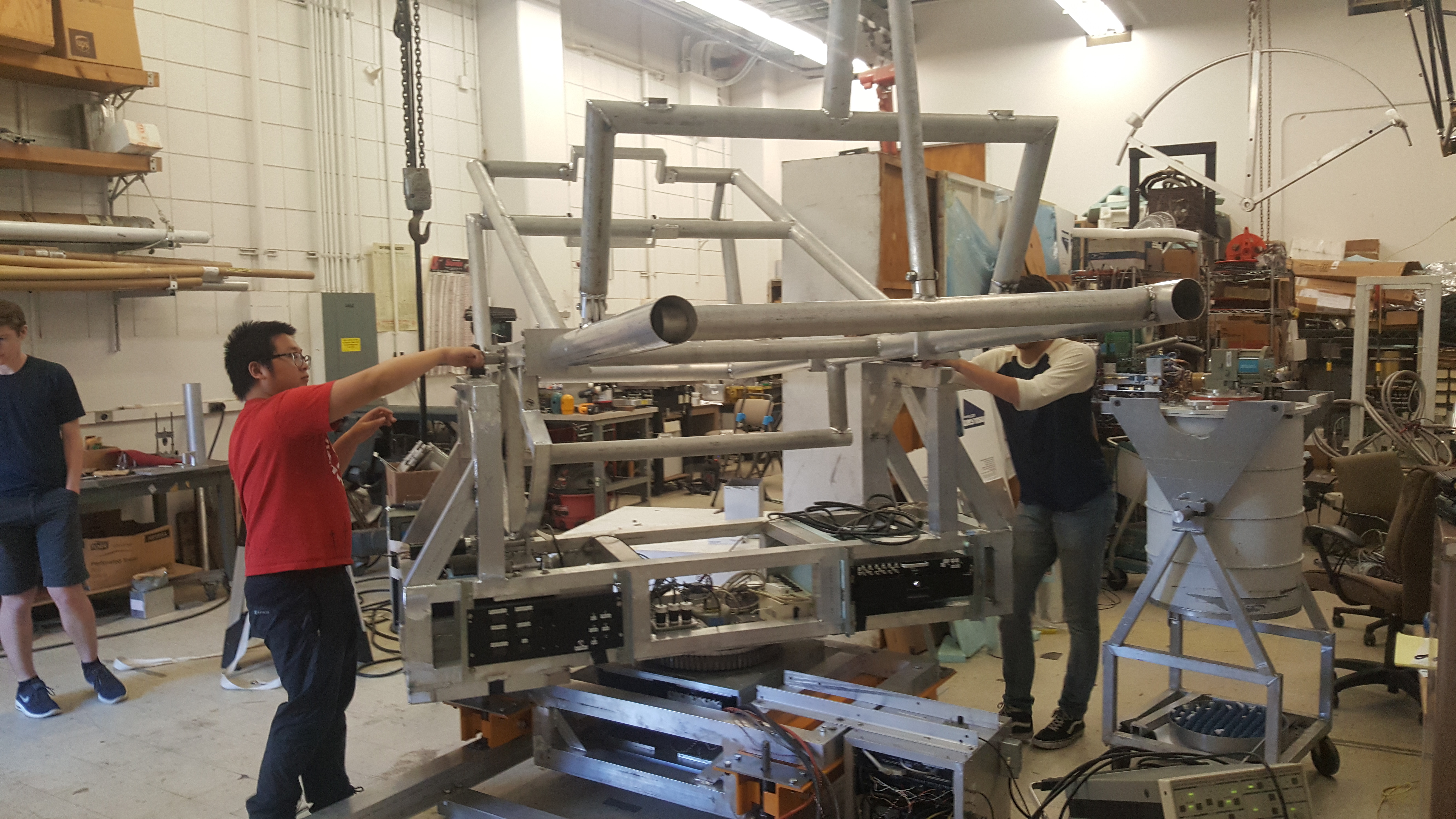
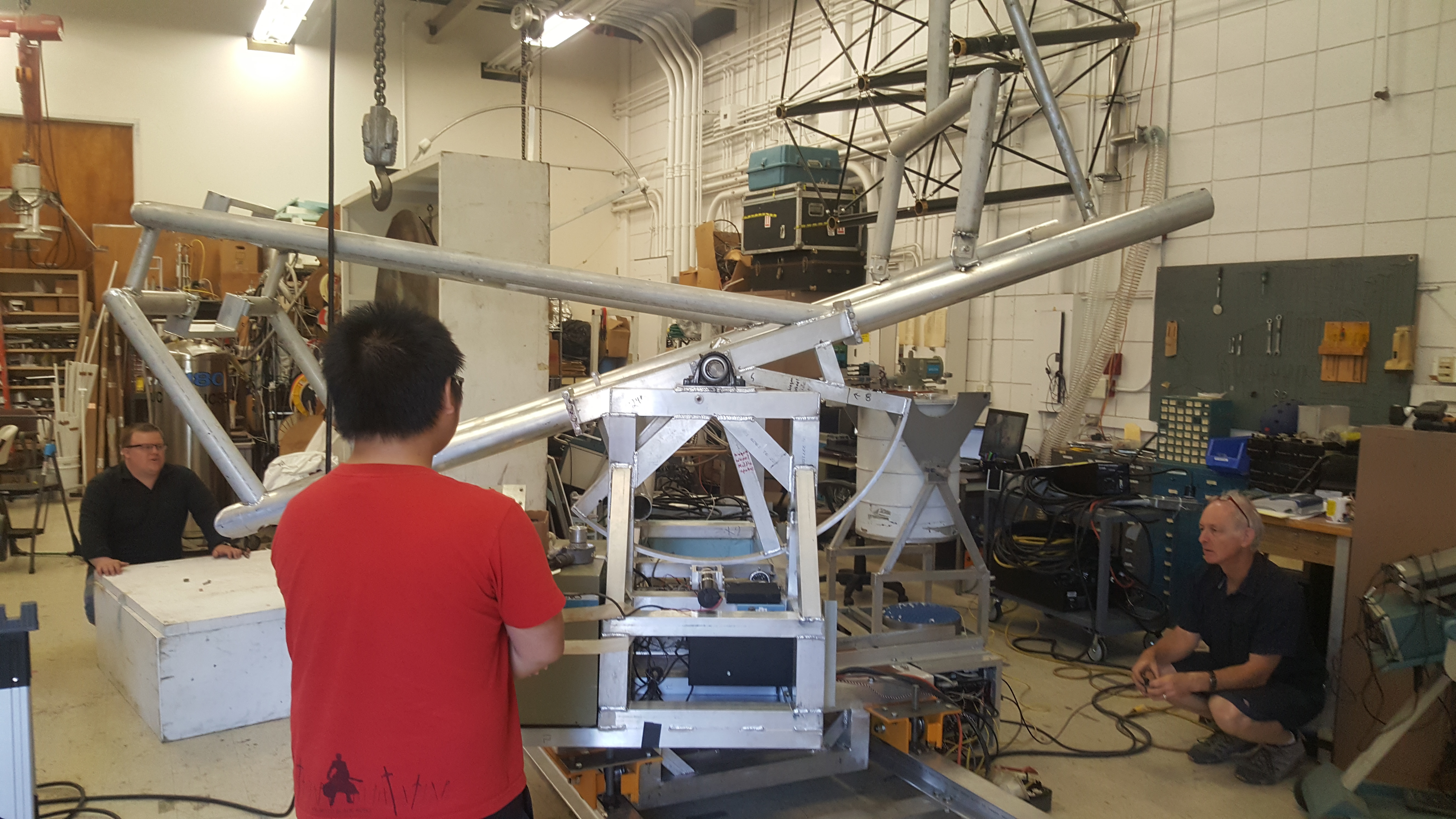
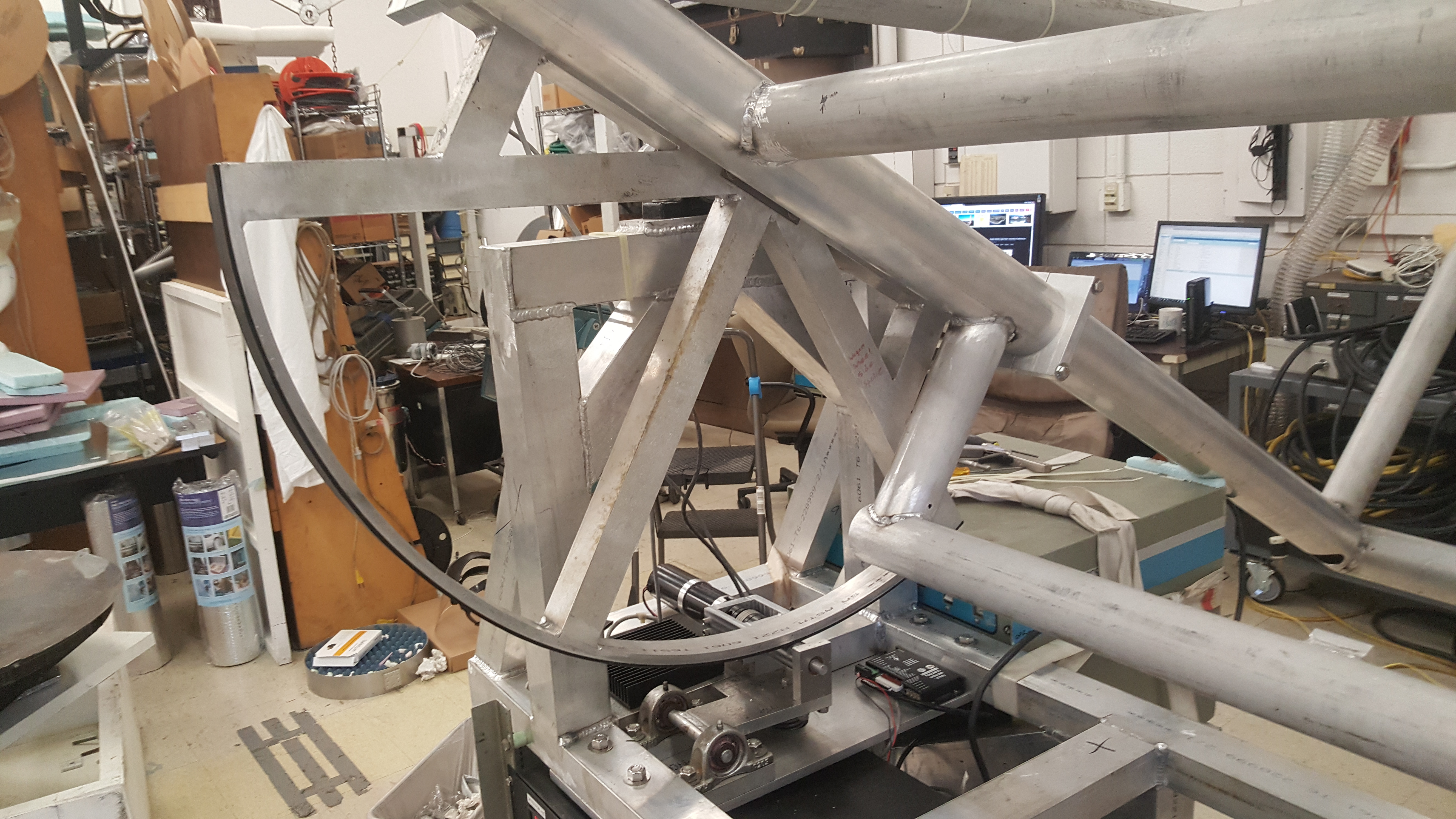
Elevation Drive
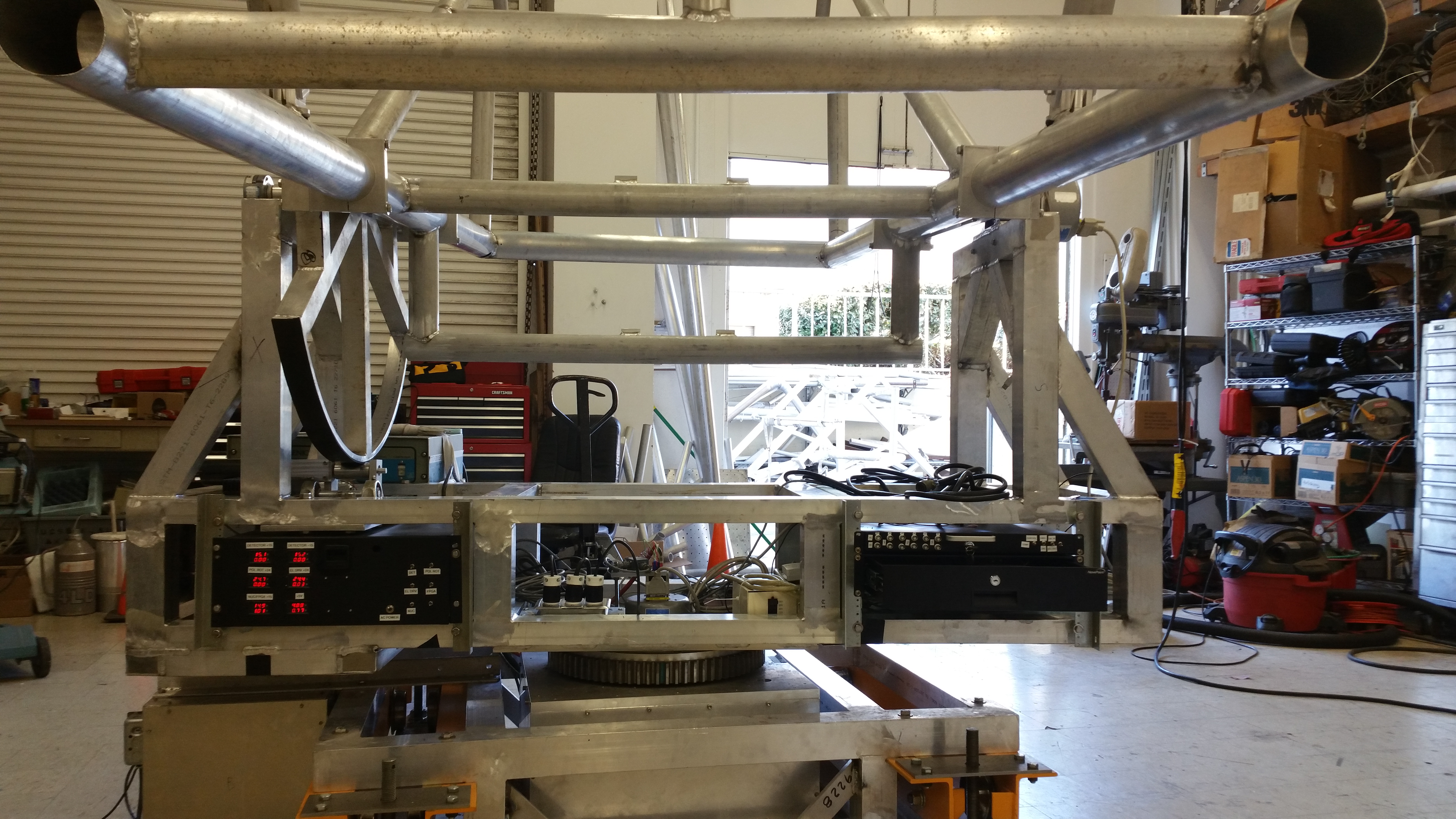
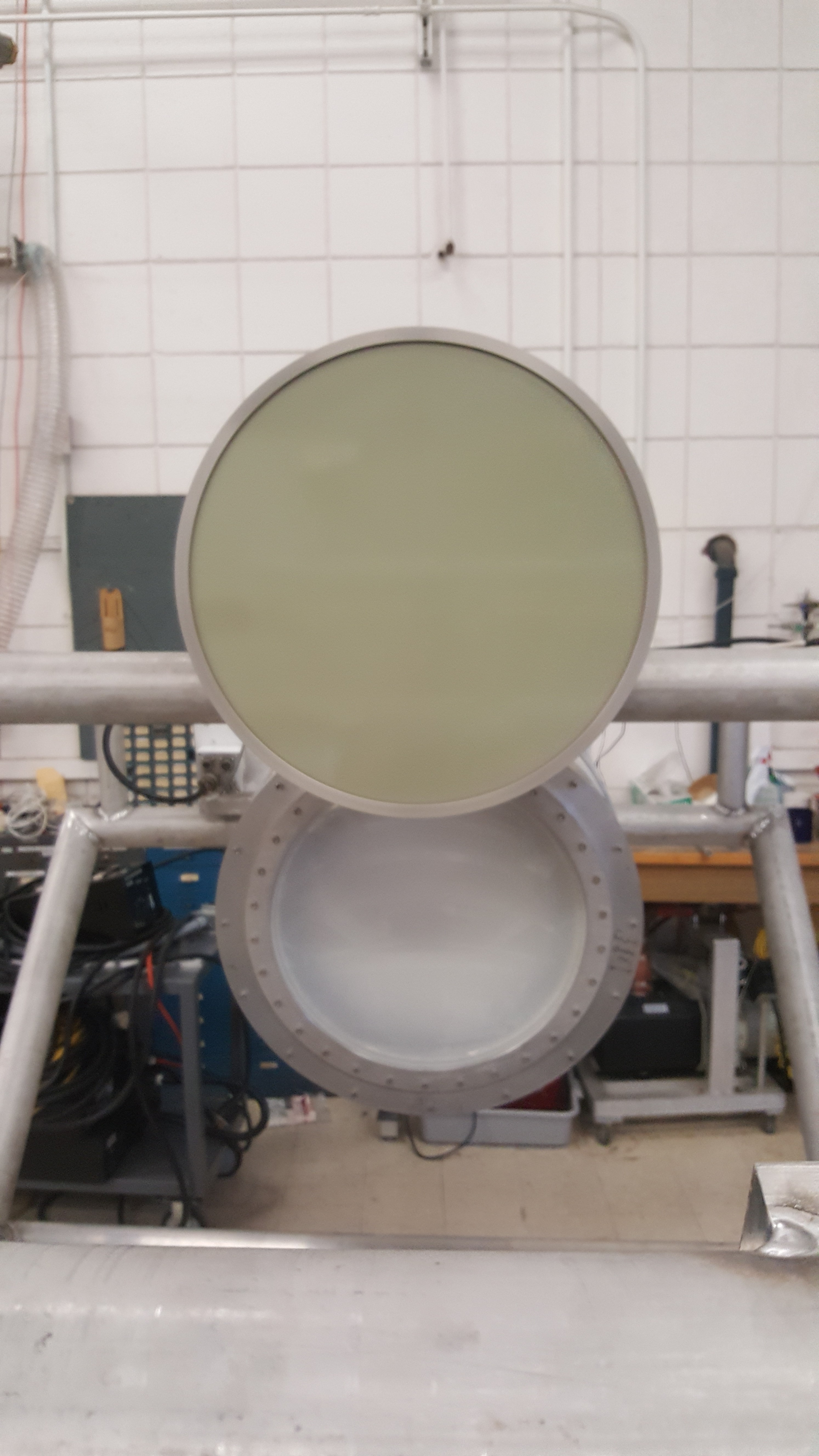
Polarizing Grid
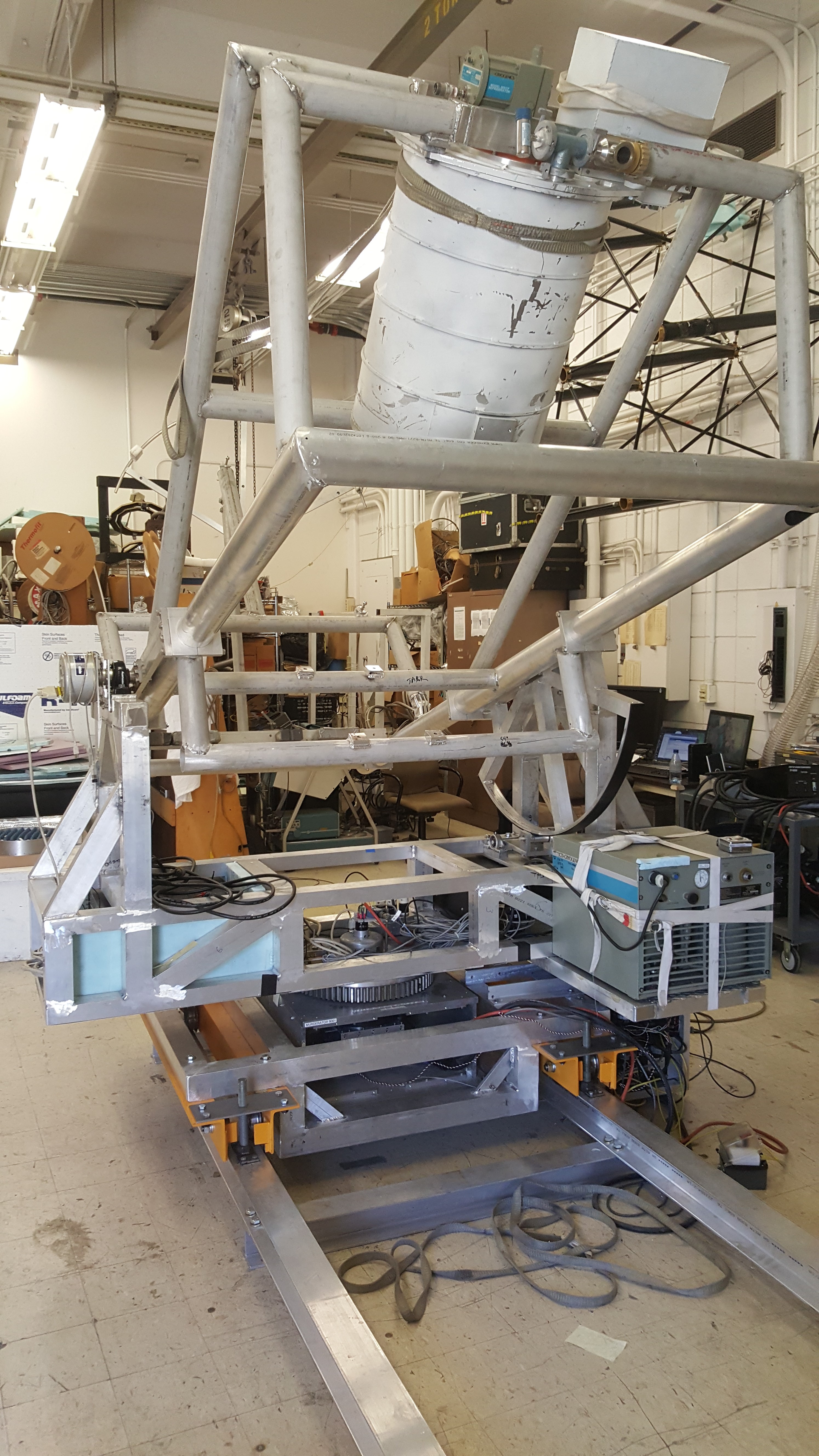
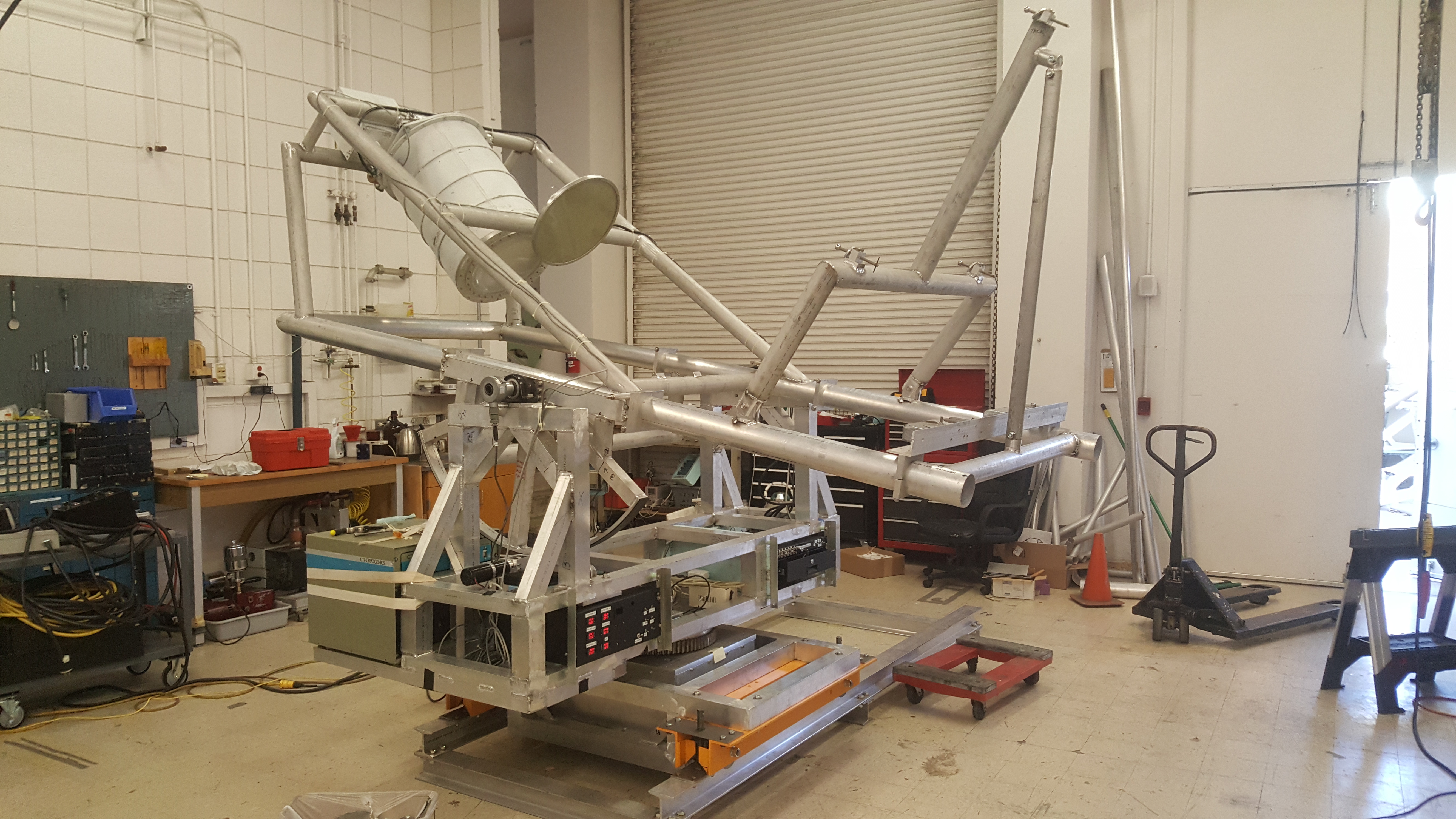
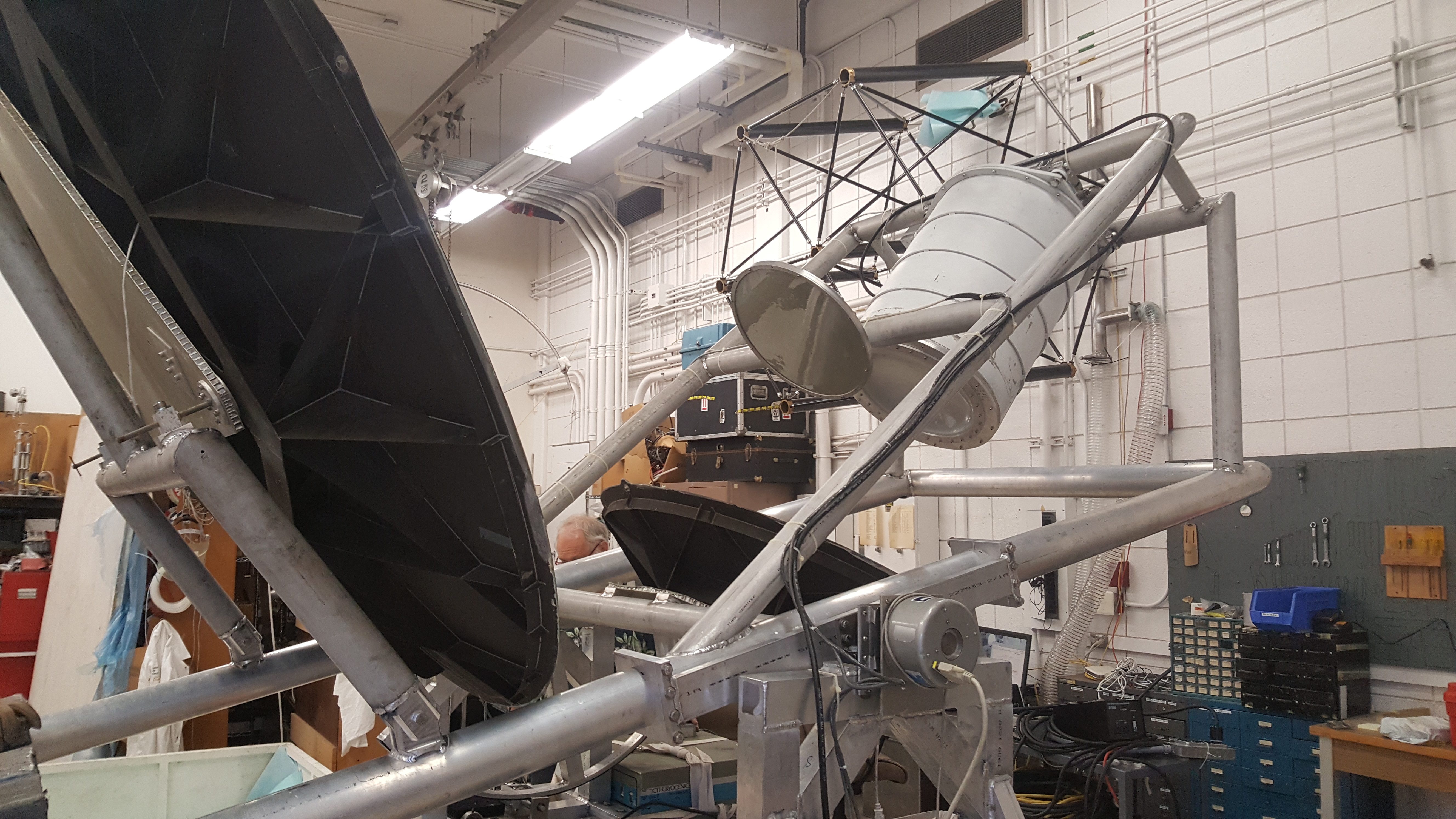
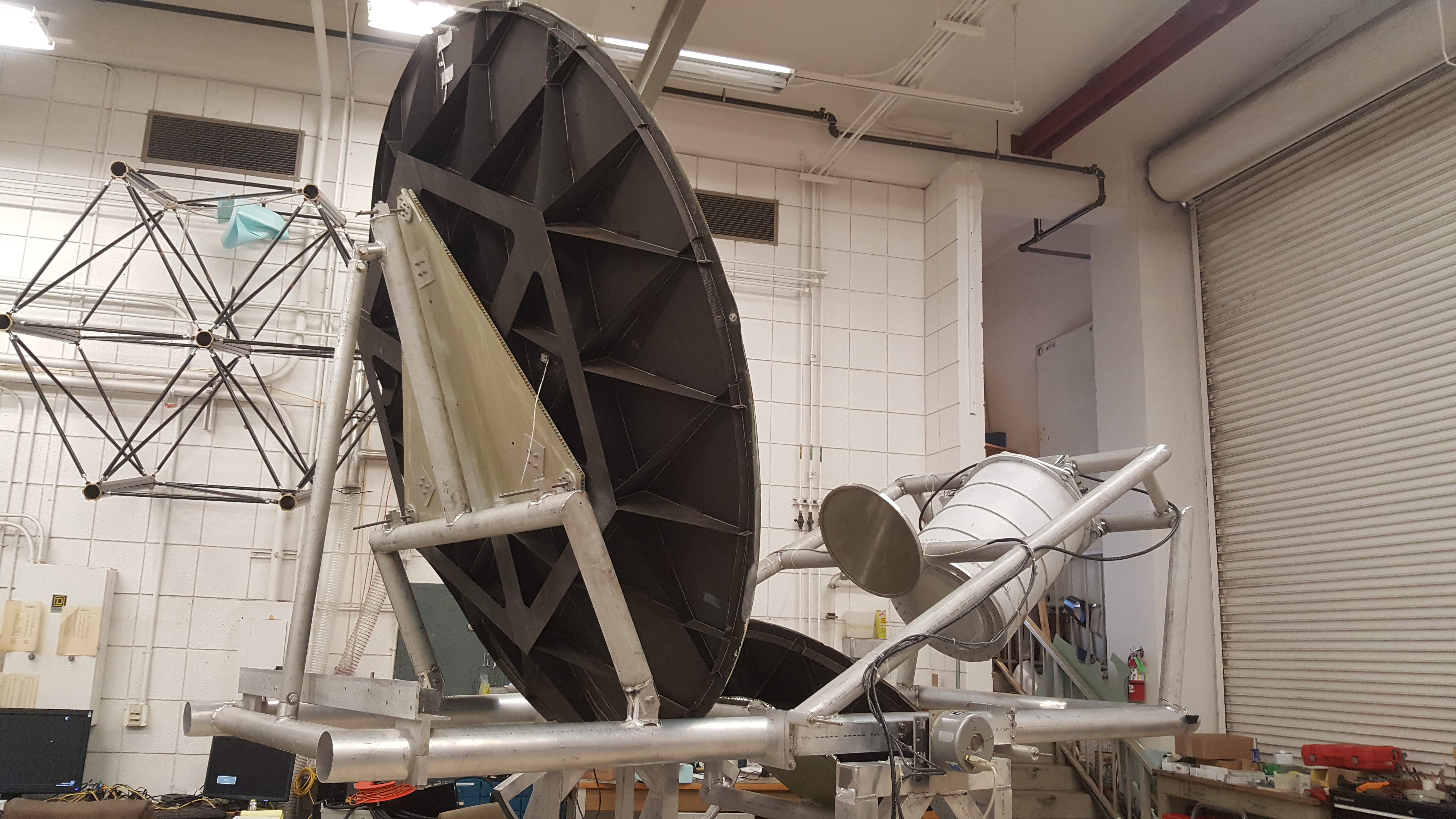
20170725_143931
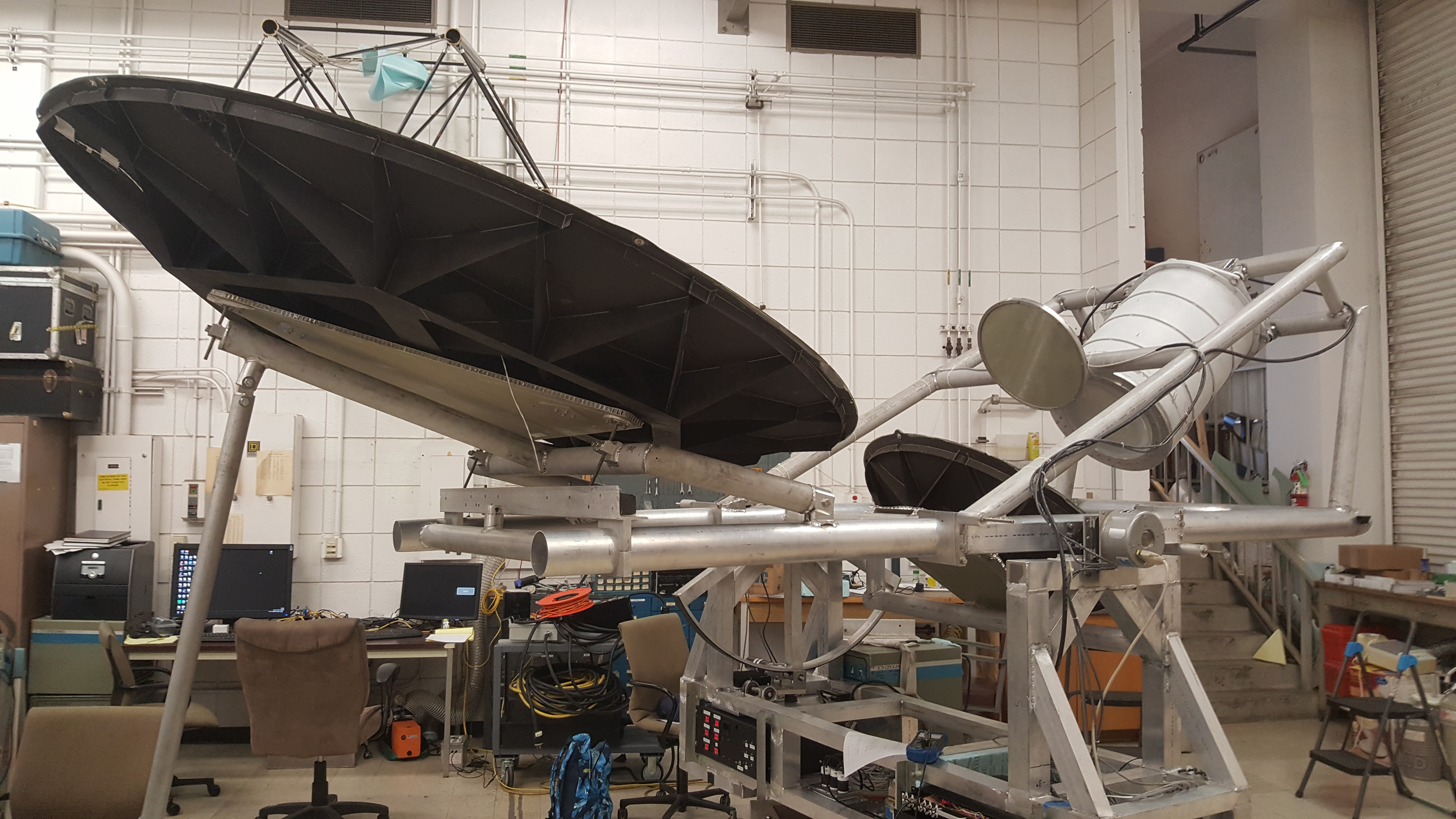
20170725_144144
Optical Modeling
An important problem will be accurately modeling our telescope response (radiation) pattern so as to reduce side-lobe contamination and improve systematics. Side-lobes (or back-lobes) are the lobes of the far-field radiation pattern which are outside the main-lobe (where you are pointing) and are caused by diffraction around the optical components of the telescope. This can be a serious issue when your measurements are being dominated by bright sources located away from where you are pointing, leading to high noise sky maps. Fully understanding this response pattern is then essential to knowing what in your measurements is erroneous. The response pattern can also be made less complicated by reducing side-lobe contamination initially with a Baffle, which acts as an extension to the primary mirror. Therefore optimizing this baffle design is an essential component of the GreenPol optics.
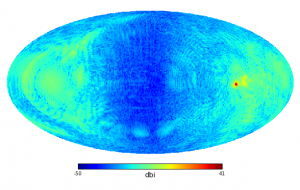
Fig 1. Telescope response pattern with no baffle or ground/side shielding.
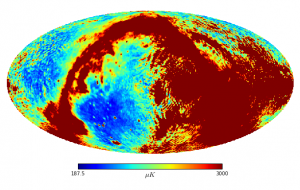
Fig 2. No baffle or ground/side shielding, telescope response to Planck 10 GHz T signal + 5800 K sun on 7/15/2017 at 12 am
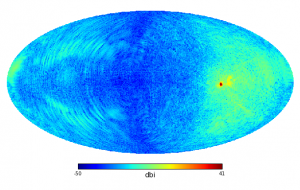
Fig 3. Telescope response pattern with baffle and ground,back,and side shielding.
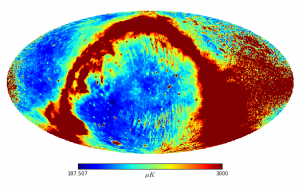
Fig 4. Full shielding + baffle, telescope response to Planck 10 GHz T signal + 5800 K sun on 7/15/2017 at 12 am
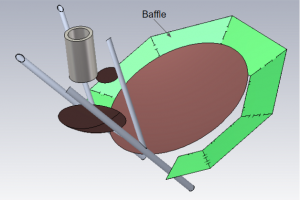
Fig 5. Optical Model of GreenPol telescope with addition of Baffle
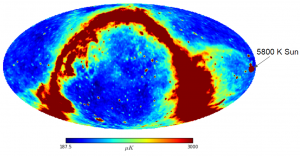
Fig 6. Full shielding + baffle, main-lobe (~3 FWHM from beam center) only response to Planck 10 GHz T signal + 5800 K sun on 7/15/2017 at 12 am
What you are seeing in figure 2 is the convolution between the response pattern map in figure 1, and a 5800 K sun added to the Planck 30 GHz temperature signal extrapolated to 10 GHz, i.e. an estimate of how our telescope would map the sky given these components. Side-lobe pickup of the sun, whose location can be seen in the (side-lobe removed) map of figure 6 to the far right, completely dominates measurements over a large portion of the sky, leaving little room for low noise measurements. With the addition of a baffle and additional shielding, this situation is improved marginally, as can be seen in figures 3 and 4. While a large portion of the sky is still dominated by side-lobe pickup of the sun, there are now more regions of the sky accessible to lower noise measurements. These simulations illustrate the strong need for both an optimized baffle design, which reduces the complexity of our response pattern, and accurate modeling of our response pattern, which is necessary to effectively remove side-lobe contamination in our data and reduce systematics in our final sky maps.
Team
Institution | PhD Scientists | Staff | Graduate Students | Undergraduate Students |
---|---|---|---|---|
University of California at Santa Barbara | Philip Lubin | Nic Rupert | Ari Kaplan | Aryan Zaveri |
Peter Meinhold | Lianghao Chen | |||
Natalie LeBaron | ||||
Paul Park | ||||
Pierre Thibodeux | ||||
Scott Marino | ||||
Zijian Ding | ||||
University of Oslo | Hans Kristian Eriksen | Unni Fuskeland | ||
Ingunn Kathrine Wehus | Harald Thommesen | |||
Niels Bohr Institute | Per Rex Christensen | |||
Sebastian Domenico von Hausegger | ||||
Hao Liu | ||||
Pavel Naselsky | ||||
University of California at San Diego | Andrea Zonca |
Funding
Funding for this project comes from the Niels Bohr Institute and the Villum Foundation
Contact
For more information please contact:
Philip Lubin
Physics Professor
Experimental Cosmology Group
Department of Physics,
University of California,
Santa Barbara, CA 93106-9350
Peter Meinhold
Research Physicist
Experimental Cosmology Group
Department of Physics,
University of California,
Santa Barbara, CA 93106-9350
Ari Kaplan
Graduate Student
Experimental Cosmology Group
Department of Physics,
University of California,
Santa Barbara, CA 93106-9530